All published articles of this journal are available on ScienceDirect.
Monitoring the Level of 14C-Labelled Selegiline Following Oral Administration
Abstract
Background:
Selegiline [(-)-deprenyl] is widely used for the treatment of Parkinson’s disease in humans.
Objective:
Time-dependence of tissue distribution of selegiline following per os administration to rats.
Method:
Oral administration of radiolabeled selegiline to rats resulted in a pattern of tissue distribution similar to that following intraperitoneal injection. Analyses were done using both reversed-phase HPLC and also by counting radioactivity in various body compartments of rats.
Results:
As a consequence of oral administration of 30 mg/kg of selegiline, its level in the stomach was extremely high (179.57 µg/g tissue through 54.67 µg/g at 15 min to 120 min), that is one magnitude higher than that in the serum level. High selegiline concentrations were also detected in the lacrimal glands (7.45 µg/g), kidneys (6.87 µg/g), livers (6.01 µg/g) and lungs (3.47 µg/g) after 30 minutes of application, which were higher than after intraperitoneal injections.
Conclusion:
The relatively high tissue levels remained for 120 min monitoring. Selegiline levels in the brain (1.69 µg/g) and in the testes (1.88 µg/g) were also considerably higher than following intraperitoneal administration during the entire period of observation (15 to 120 min).
1. INTRODUCTION
Selegiline [(-)-deprenyl hydrochloride] (Fig. 1) is widely used for the treatment of Parkinson’s disease in humans [1]. It is a specific and irreversible inhibitor of the monoamine oxidase B enzyme when used in therapeutic doses [2-4].
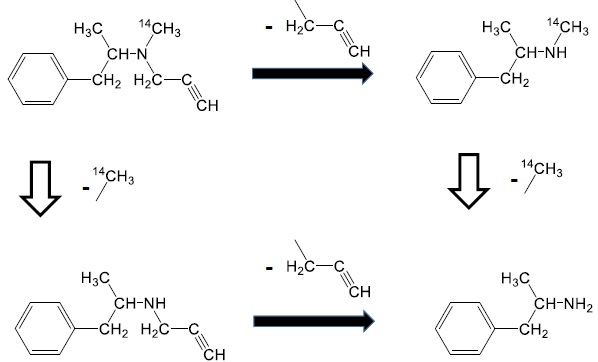
Ehringer and Hornykiewicz [5, 6] detected that the dopaminergic tone of the human brain progressively declined with age and also in certain diseases. This phenomenon is characteristic of Parkinson’s disease. Selegiline exerts its effect in the dopaminergic system of the brain counterbalancing this decline by multiple mechanisms. Selectively and irreversibly inhibiting the MAO enzyme, selegiline is responsible for the inhibition of the degradation of dopamine [3], moreover, selegiline inhibits the high-affinity reuptake of biogenic amines (such as dopamine) [7, 8] and also facilitates dopamine release from the corpus striatum [9]. Acute selegiline treatment produces either no or only moderate effect [10]. Following chronic administration, a definite increase in both the dopamine content and the potassium-induced dopamine release in the brain tissue could be observed [9].
In addition to the therapeutic use of selegiline in Parkinsonism, its further beneficial effects have also been published. Knoll, et al. [11-13] found an increased life span of rats as a result of chronic treatments with selegiline and p-fluoroselegiline [14]. Independent research groups [15-17] confirmed Knoll’s results, and claimed that longevity of rats was originated from the inhibition of oxidative enzymes by selegiline [17, 18]. Knoll and Dalló published the positive correcting effect of selegiline and p-fluoroselegiline on sluggish sexual behavior of male rats [14, 19].
Definite incorporation during drug distribution takes place in silent binding sites (such as serum proteins) [20, 21] and active binding sites, such as receptors and various receptor-like binding sites, such as special sites including enzymes [22]. Active binding sites can be the target of drug for drug discovery [23]. The effect of drugs serves to locate their binding site(s). Our recent publications definitely suggested incorporation of selegiline in the brain and testes following i.p. administration [24, 25]. Similarly, deuterated fluoroselegiline showed incorporation and served for PET studies of monoamino oxidase B in the cynomolgus monkey brain [26]. In pharmacological experiments on the distribution and metabolism of selegiline, intravenous and intraperitoneal injections are administered in order to avoid the first-pass effect of the liver. However, the majority of human treatments prefer oral administration to injection if it is not contraindicated. This paper is devoted to determine time-dependence of tissue distribution of selegiline following per os administration to rats.
2. MATERIALS AND METHODS
2.1. Materials
2.1.1. Chemicals and Solvents
Selegiline hydrochloride (Fig. 1) was the kind gift of Chinoin Pharmaceutical and Chemical Company, Budapest, Hungary (its recent name is: Chinoin Sanofi-Aventis, Budapest, Hungary). Radiolabeled selegiline (14C-methyl) was synthetized in the Isotope Institute (Budapest, Hungary) with a specific activity of 1.851 MBq/mg. Sodium citrate, disodium phosphate, octane sulfonic acid sodium, Na2-EDTA, hydrogen peroxide and HPLC grade acetonitrile were purchased from Sigma-Aldrich Kft. (Budapest, Hungary).
Soluene-350 and Optiphase scintillation cocktails were purchased from Perkin Elmer (PER-FORM Hungária Kft, Budapest, Hungary).
2.2. Animals
Male Wistar rats were supplied by Toxicoop (Budapest, Hungary) weighing 195 – 205 gram. The experimental protocol was approved by the ethical committee of ANTSz (Budapest, Hungary) with a permission number 1810/003/2004. All experimental conditions conformed to 86/509/EEC regulation.
Rats (in each group n = 3) were orally (per os) treated with 30 mg/kg 14C-labeled selegiline dissolved in saline. The rats were sacrificed following 15, 30, 60 and 180 minutes of treatment. Blood, CSFs, urine, faeces, the brains, lacrimal glands, salivary glands, testes, kidneys, livers, lungs, hearts, stomachs, eyes and cochleas were isolated or dissected. The samples were kept at -80 ºC until analysis.
2.2.1. Sample Preparation
The samples were homogenized in 0.3 M of perchloric acid using Ultra Turrax T25 homogenizer (IKA Labortechnik, Staufen, Germany) at 20,000 rpm for 30 sec at room temperature. Homogenates were centrifuged at 14,000 rpm for 20 min at 4 ºC in an Eppendorf centrifuge, (A. Hettich, Tuttlingen, Germany) and supernatants were used for selegiline determination by RP-HPLC.
Calibration curve was constructed with triple injections of 6 spiked standard samples from 0.1 µg/ml through 5 µg/ml selegiline (Fig. 2). Selegiline levels in the samples were calculated by the mean of the results of triple injections.
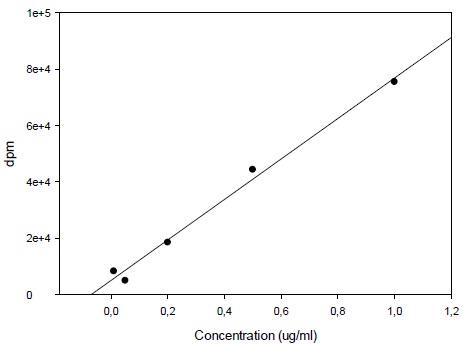
2.2.2. HPLC Determination of Selegiline Content
The set-up for HPLC was purchased from ABL&E Jasco (Budapest, Hungary). It consisted of a JASCO DG-2080-54 Degasser, JASCO PU-1580 Pump, JASCO AS-2057 Plus Automatic Sample Injector, an Intro EC detector (Antec, Leyden, the Netherlands), a JMBS Hercule 2000 Chromatography Interface and a Borwin 1.50 Controller and Evaluation Software.
The stationary phase was a Zorbax Rx-C18 (4.6 mm x 250 mm x 5 µm) column purchased from Kromat Kft (Budapest, Hungary).
The mobile phase was a 2:8 (v/v) mixture of acetonitril: aqueous buffer at pH 3.7, while the buffer was the solution of 10 g citric acid, 10 g trisodium phosphate, 100 mg OSA, dissolved in 600 ml HPLC quality of water, which was completed to 800 ml. Temperature of the column was kept at 35 ºC and analyses were done using 1 ml/min flow rate. An injection volume of 75 μl was applied.
An Intro EC detector using amperometric detection mode at 0.95 V was used and the total range was 2 nA.
2.3. Determination of Radioactivity in Various Samples
2.3.1. Treatment of Tissue Samples
About 100 mg of each liver, brain, lacrimal glands, salivary gland and testes were transferred into 20 ml scintillation vials. 1.5 ml Soluene-350 was added to each sample, kept at a 60 ºC water bath for 90 minutes and was then cooled down to room temperature. Afterwards, 0.2 ml of 30% hydrogen peroxide was given in two portions (that is twice 0.1 ml) and was shaken well after each portion. The samples were kept at room temperature for 30 minutes, followed by keeping at 60 ºC for 30 minutes. After cooling down to room temperature, 10 ml scintillation cocktail was given to each sample and radioactivity counting was performed after 12 hours in a Beckman LS-5000TA counter. The treatment of serum and urine samples was similar except that 50 µl of the samples was taken. Moreover, 0.5 ml isopropanol was also given to the samples following treatment with 30% hydrogen peroxide and provision of the scintillation cocktail.
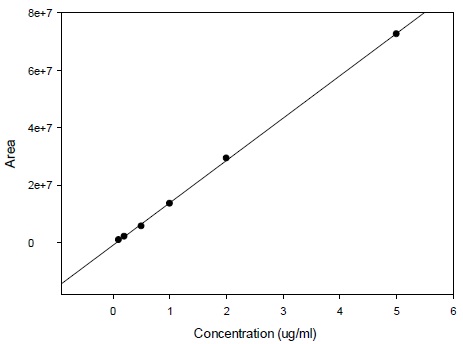
3. RESULTS
3.1. Calibration for 14C-Deprenyl Content
Calibration curve for the determination of radioactivity is given in Fig. (2). Fig. (3) gives an HPLC determined calibration curve of non-metabolized selegiline. Fig. (4) triplet gives a representative chromatogram of selegiline in testis. Fig. (4a) shows the chromatogram of blank (untreated) testis. Fig. (4b) shows the chromatogram of testis spiked with selegiline and Fig. (4c) gives the chromatogram of testis from a selegiline-treated rat.
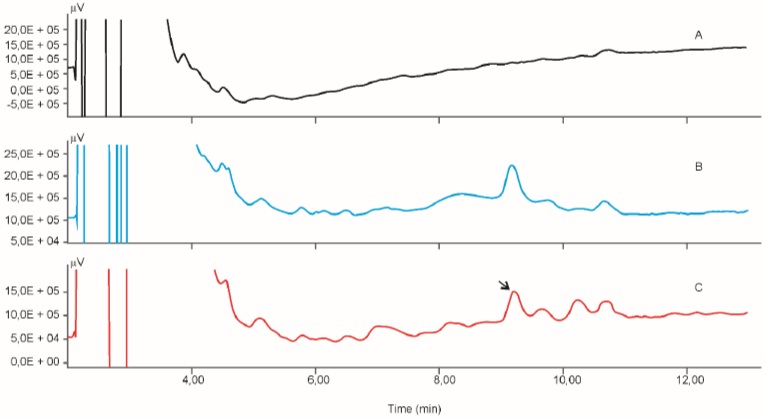
Table 1 provides the results of radioactivity determinations in the tissues and body fluids. Radioactivity is expressed in µg/g or µg/ml, based on dpm/g wet tissue or dpm/ml body fluid. Radioactivity indicates the presence of the metabolically intact selegiline plus its major metabolite and the level of (-)-methamphetamine (Fig. 1).
Tissue or Body Fluid | Selegiline (or methamphetamine) level, µg/ml or µg/g following 15 min | Selegiline (or methamphetamine) level, µg/ml or µg/g following 30 min | Selegiline (or methamphetamine) level, µg/ml or µg/g following 60 min | Selegiline (or methamphetamine) level, µg/ml or µg/g following 120 min |
---|---|---|---|---|
Serum | 7.348 | 3.844 | 5.123 | 8.541 |
Brain | 5.842 | 2.258 | 2.234 | 4.266 |
Cochlea | 3.040 | 3.793 | 4.825 | 6.571 |
Eyes | 2.123 | 1.364 | 2.850 | 2.245 |
Lacrimal gland | 15.172 | 7.617 | 9.636 | 19.284 |
Salivary gland | 5.764 | 3.332 | 5.080 | 6.414 |
Testis | 3.264 | 2.354 | 2.259 | 3.923 |
Kidney | 14.461 | 7.277 | 6.489 | 12.392 |
Liver | 49.323 | 26.842 | 32.208 | 81.143 |
Lung | 11.051 | 4.495 | 5.282 | 8.444 |
Heart | 5.678 | 2.879 | 2.694 | 4.978 |
Stomach | 263.229 | 98.048 | 106.372 | 77.322 |
Urine | --- | 474.172 | 90.512 | 769.814 |
Faeces | 1.240 | 0.809 | 0.782 | 3.727 |
Table 2 provides the non-metabolized selegiline content of body fluids and tissues of rats on the basis of HPLC determinations. Triplicate determinations were carried out. The tissue level of selegiline in the stomachs was extremely high at each sample (15, 30, 60 and 120 mins) as selegiline was administered orally and the decline during the experimental period indicates its transfer into the ileum. High contents of selegiline were detected in the lacrimal glands, the kidneys, the livers, and in the lungs at all of the time points detected.
Pharmacokinetics shows the upmost increase of selegiline in the livers, as well as a definite (but moderate) increase in the lacrimal glands, cochleas and kidneys.
Tissue or Body Fluid | Selegiline level, µg/ml or µg/g following 15 min | Selegiline level, µg/ml or µg/g following 30 min | Selegiline level, µg/ml or µg/g following 60 min | Selegiline level, µg/ml or µg/g following 120 min |
---|---|---|---|---|
Serum | 2.06 | 1.51 | 1.66 | 2.20 |
Brain | 1.69 | 1.45 | 1.65 | 1.54 |
CSF | 1.50 | 1.96 | 1.64 | 1.53 |
Cochlea | 1.25 | 1.18 | 1.37 | 1.83 |
Eyes | 1.92 | 1.85 | 3.82 | 5.68 |
Lacrimal glands | 9.88 | 7.45 | 3.75 | 5.22 |
Salivary glands | 2.27 | 2.49 | 1.90 | 2.24 |
Testis | 2.01 | 1.88 | 1.86 | 2.03 |
Kidney | 9.81 | 6.87 | 2.48 | 4.60 |
Liver | 6.61 | 6.01 | 2.51 | 2.33 |
Lung | 4.56 | 3.47 | 3.62 | 3.99 |
Heart | 2.26 | 1.85 | 1.89 | 2.22 |
Stomach | 179.57 | 76.16 | 89.69 | 54.67 |
4. DISCUSSION
The chemical structure of radiolabeled selegiline (Fig. 1) shows that the N-methyl group contains the source of radioactivity in the form of 14C. However, the major metabolite of selegiline is methamphetamine which maintains the radioactivity keeping the 14C-methyl group as well [27, 28].
In Ehrlich's beloved Latin terminology: Corpora non agunt nisi fixata (agents only work when they are bound) [29]. The key–lock principle led to the side-chain theory of antibody formation, which became a cornerstone of the newly-emerging discipline of immunology recognized by the Nobel Prize. Ehrlich formulated his ideas in chemical terms, speaking of “chemical affinities” in biological processes (e.g. toxin/antitoxin or antigen/antiserum complexes, “magic bullets” seeking specific targets). Some three decades of his experience were epitomized in his maxim, “Corpora non agunt nisi fixata, i.e. a substance is not (biologically) active unless it is “fixed” (bound by a receptor)”. Penetration of selegiline into the testis is thereby of basic importance from the point of view of MAO enzyme inhibition.
Roberge, et al. [30] reported the dependence of activity of human seminal plasma on its level of monoamine oxidase. As early as thirty years ago, an observation was made according to which the MAO enzyme was present in the seminal plasma and a higher MAO activity was detected in infertile men than in fertile ones. Monoamine oxidase (MAO) content and activity in the testes show changes during sexual differentiation [31, 32]. MAO inhibitors affect the copulatory behavior of male rats [33]. At the same time, there is a blood-testis barrier and its function is to select penetration of both xenobiotics and endogenous compounds [34-37].
A recent publication by Mihalik, et al. [38] claims the favorable effect of selegiline on the increase in the number and motility of sperms of male rats following chronic intraperitoneal treatment with selegiline.
Pharmacokinetics of orally given selegiline are subjected to the alterating influence of its first-pass effect. Oral administration of various drugs used in humans is generally preferred to any other (i.p., i.v., s.c.) except in the special cases. This is the reason why oral treatments are carried out. However, pharmacology can use either i.p. or i.v. administration to avoid the first-pass effect of the drug. This method has been used and reported in our preliminary publications [9, 24, 25, 27]. However, to monitor the pharmacokinetics related to the use of selegiline in humans, oral administration should be applied. Certain fluctuations in the selegiline levels in the serum, brain, etc. must be the formation and completion/saturation of the first-pass effect of the liver.
CONCLUSION
A high deprenyl level was found in the stomachs, kidneys, lacrimal glands, livers and lungs after 15 minutes of administration, indicating the site of absorption as well as the major excretion routes. The relatively constant selegiline levels in the brain and testes show the sites where selegiline is bound by the monoamine oxidase enzyme. This interaction can cause an inhibition of this enzyme resulting in an elevated number and motility of sperms.
CONFLICT OF INTEREST
The authors confirm that this article content has no conflict of interest.
ACKNOWLEDGEMENTS
Kalász Teaching and Research Co (Budapest, Hungary) and the Hungarian National Granting Agency have financially sponsored this project with OTKA No. 100155 grant. Cooperation with and advice by Dr. Tibor Zelles and Mr. János Horváth are highly appreciated.
REFERENCES
[PubMed Link]
[PubMed Link]
[PubMed Link]
[PubMed Link]
[PubMed Link]
[PubMed Link]
[PubMed Link]
[PubMed Link]
[PubMed Link]
[PubMed Link]
[PubMed Link]
[PubMed Link]
[PubMed Link]
[PubMed Link]
[PubMed Link]
[PubMed Link]
[PubMed Link]
[PubMed Link]
[PubMed Link]
[PubMed Link]
[PubMed Link]
[PubMed Link]
[PubMed Link]
[PubMed Link]
[PubMed Link]
[PubMed Link]
[PubMed Link]
[PubMed Link]
[PubMed Link]
[PubMed Link]