All published articles of this journal are available on ScienceDirect.
Advancing Alzheimer's Therapy with Moringa oleifera: Bioactive Insights, Mechanistic Pathways, and Strategies for Efficacy and Standardization
Abstract
Alzheimer’s disease (AD) represents a devastating condition that profoundly impacts the quality of life for both affected individuals and their families. Currently, there is no viable treatment for AD. Conventional drugs for AD provide symptomatic relief by temporarily improving cognitive function or slowing the progression of specific symptoms. These medications do not stop or reverse the underlying neurodegeneration, offering only modest benefits in managing the disease, and often come with unpleasant side effects, making adherence challenging for patients. Natural treatments made from plants have drawn interest for their potential to lessen AD symptoms while having a lesser side-effect profile. Among these, Moringa oleifera (M. oleifera), also referred to as the “miracle tree,” has gained attention for its wide range of health benefits, including antioxidant, anti-inflammatory and neuroprotective properties. By delving into the bioactive compounds underpinning its mechanisms of action, exploring M. oleifera through various omics technologies, and addressing the challenges associated with its standardization, this review provides an in-depth analysis of its potential in AD therapy. This review also highlights the need for further research to unlock the full spectrum of factors that could enhance the efficacy of M. oleifera in combating AD by bridging its phytochemical properties with practical therapeutic applications. Therefore, understanding and harnessing the full therapeutic potential of M. oleifera could pave the way for more effective interventions in AD management.
1. INTRODUCTION
The World Health Organization (WHO) declared dementia a public health priority in May 2017 and approved the Global action plan on the public health response to dementia for the year 2017-2025 [1]. The plan outlines a detailed action plan to raise public awareness about dementia. According to research, more than two-thirds of Alzheimer's patients live in low and middle-income nations [2]. In 2020, approximately 55 million people worldwide were living with dementia. The world's population of elderly people is growing, and the number is predicted to rise to 78 million by 2030 and 139 million by 2050 [3].Alzheimer’s Disease (AD) is a progressive neurodegenerative condition characterized by memory loss and a decline in daily life functioning. It predominantly affects individuals aged 65 and older and is the most prevalent form of dementia. While its precise cause remains unknown, several risk factors, such as advancing age, family history, genetics, and lifestyle, are known to contribute to its development [4]. Symptoms of AD manifest gradually over several years, with varying stages, including pre-clinical, mild, and probable dementia [5]. Early signs often involve memory lapses, such as forgetting recent information. In the mild dementia stage, memory problems worsen, and individuals may experience difficulty speaking fluently and exhibit personality changes such as increased aggression. In advanced stages, symptoms intensify, encompassing issues like urinary incontinence and weight loss due to eating difficulties.
The development of AD is driven by a complex interaction of genetic, environmental, and molecular factors, each contributing to the disease's progression in unique ways. The hallmark of AD includes the accumulation of abnormal proteins in specific brain regions involving tau tangles and amyloid plaques [6]. Amyloid beta peptide (Aβ) is central to plaque formation and neurofibrillary tangles caused by tau protein abnormalities. Aβ arises when amyloid precursor protein (APP) undergoes cleavage by enzymes, particularly β-secretase. The resulting Aβ peptides tend to clump together, forming plaques that disrupt communication between brain cells, leading to synaptic loss, neuron death, and brain shrinkage [6]. Mutations in APP worsen this process by boosting the production of Aβ, contributing to familial forms of AD [7]. Notably, Aβ peptides are highly prone to aggregation, progressing from small oligomers to large fibril deposits in the brain, ultimately contributing to the progression of AD.
Meanwhile, tau protein becomes hyperphosphorylated, forming tangles that impair neuronal function and contribute to cognitive decline.Beyond amyloid-βf and tau, other factors like neuroinflammation, oxidative stress, and mitochondrial dysfunction play significant roles in AD. Activated microglia and astrocytes release inflammatory cytokines that worsen neuron damage, while oxidative stress weakens cellular function, accelerating neurodegeneration. The disruption of neurotransmitter systems, such as those involving acetylcholine, glutamate, dopamine, and serotonin, also contributes to the cognitive and behavioural symptoms observed in AD. These interconnected mechanisms underline the complexity of the disease, highlighting the need for therapies that address multiple pathways to slow or stop the progression of AD.
Therapeutic approaches for AD today majorly emphasize relieving symptoms rather than putting efforts to deal with the root cause of the disease. Donepezil, rivastigmine, and galantamine are the most used drugs that belong to cholinesterase inhibitors, and their target is to improve cognition by increasing levels of acetylcholine in the brain. There is also another set of drugs, which includes memantine, which is an NMDA receptor antagonist helpful in regulating glutamate activity to protect neurons from excitotoxic damage [8]. However, these pharmacotherapies provide only minor and transient clinical benefits without preventing or reversing disease progression, occasionally associated with side effects that contribute to patient noncompliance. Despite ongoing efforts, disease-modifying treatments targeting amyloid-β and tau proteins have faced significant setbacks in clinical trials, with many candidates failing to reduce cognitive decline or improve quality of life. This highlights a major limitation in current AD therapies, which focus primarily on managing symptoms rather than halting the underlying neurodegenerative processes. Given the limitations of existing treatments, there is a need for innovative therapeutic approaches that address the multifaceted nature of AD, which includes neuroinflammation, oxidative stress, and neuronal loss, resulting in more efficient and effective treatment alternatives on a larger scale. While targeting amyloid and tau pathology remains a major focus in AD research and treatment, several alternative approaches aim to address other aspects of the disease. Few studies have been carried out to explore the use of anti-inflammatory medications to reduce neuroinflammation associated with AD [9]. Several vaccines or antibodies targeting specific proteins associated with inflammation in the brain are currently being developed, such as Aducanumab, Lecanemab, Donanemab and Gantenerumab [10, 11]. Besides, senolytic therapy has recently been offered as a viable treatment for AD. Senolytic is a form of treatment that targets and eliminates senescent cells in the body [12]. Several studies found that senolytic substances may help to prevent the onset and progression of AD by removing the senescent cells in the brain [13, 14].
M.oleifera, an indigenous tree, is widely cultivated in tropical and subtropical regions worldwide, including India, Nepal, Pakistan, and Malaysia. It typically grows to a height of 25-30 feet and thrives in areas with light, sandy soil [15]. Known as a multipurpose plant [16], it offers various edible components such as leaves, seeds, pods, bark, roots, and flowers. It is also renowned for its high nutritional value and has historically utilized in diverse ways. For example, its seed oil is commonly used in hair care products [17], while roots and leaves are employed as traditional remedies for snakebites and wound healing [18]. Different parts of M. oleifera, including its seeds, roots, leaves and fruits, possess antioxidant capacities [19]. Among these, the leaves have been reported to have higher antioxidant activities due to their elevated polyphenol content [20]. Antioxidants are defined as any substance that, even at low concentrations compared to an oxidizable substrate, significantly delays or inhibits substrate oxidation [21]. M. oleifera contains natural antioxidants like quercetin, kaempferol, and chlorogenic acid. Several studies have shown that it has considerable therapeutic potential, with different parts of the plant possessing diverse pharmacological activities such as anti-inflammatory, neuroprotective, antioxidant, antihypertensive, diuretic, cholesterol-lowering, antiulcer, hepatoprotective, antibacterial, antifungal, anticancer, antitumor, antidiabetic, and antipyretic properties [22-24]. The presence of a variety of bioactive compounds in M. oleifera, such as polyphenols, flavonoids, and isothiocyanates, exhibits a range of pharmacological properties, including neuroprotection, regulation of neurotransmitter levels, reduction of oxidative stress, and modulation of inflammation. These mechanisms suggest that M. oleifera may play a significant role in addressing the underlying pathophysiological processes of AD, providing a complementary therapeutic approach alongside existing treatments. The beneficial effects of these compounds in AD are multifaceted, engaging several key pathways that collectively enhance their therapeutic potential.
The objective of this review is to critically explore the therapeutic potential of M. oleifera in the context of AD by highlighting the bioactive compounds present in M. oleifera that may contribute to its mechanism of action relevant to AD pathology, as well as discussing the extraction and analysis methods employed to isolate and characterize these compounds. Additionally, this review explores the potential of utilizing innovative omics technologies to investigate M. oleifera for managing AD. Furthermore, this review addresses the safety profile of M. oleifera. It highlights the challenges associated with its standardization, thereby emphasizing the need for further research to enhance its efficacy and optimize its therapeutic applications in AD management.
2. BIOACTIVE COMPOUNDS IN M. OLEIFERA
Numerous studies have explored the wide range of bioactive compounds in M. oleifera for their potential therapeutic benefits [22]. M. oleifera, possesses a diverse phytochemical profile comprising phenolic compounds, flavonoids, vitamins, and other bioactive constituents. These compounds impart potent antioxidant, anti-inflammatory, and neuroprotective properties to M. oleifera, rendering it a subject of intense research interest. The composition of these bioactive compounds can vary significantly depending on various factors such as part of the plant, geographical location, maturity stage, extraction method, and environmental conditions [22,25]. In this review, we discuss the main components of M. oleifera, with particular emphasis on its most studied part, the leaves. Furthermore, we highlight the key bioactive compounds and their properties most relevant to AD treatment, as summarized in Table 1.
2.1. Polyphenols
M. oleifera is rich in polyphenols, especially phenolic acids, and flavonoids, which are the dominant bioactive compounds in the plant. Phenolic acids are phenolic compounds that have one carboxylic acid group [26]. The primary phenolic acids extracted from the leaves of M. oleifera include gallic acid, chlorogenic acid, ellagic acid, and ferulic acid. The concentration of gallic acid in dried leaves has been reported as 1.034 mg/g. Meanwhile, the concentrations of chlorogenic and caffeic acids range from 0.018 to 0.489 mg/g of dry weight and 0.409 mg/g of dry weight, respectively [27].
A flavonoid is a fifteen-carbon skeleton consisting of two benzene rings linked via a heterocyclic pyrane ring. Kaempferol, myricetin, and quercetin are the main flavonoids that are found in M. oleifera leaves [22,28]. The leaves primarily contain quercetin (43.75%), with other flavonoids making up equal percentages of 18.75% each [29].
Bioactive | Pharmacological Properties | References |
---|---|---|
Phenolic acid | Antioxidant Anti-inflammatory Inhibit monoamine oxidase |
[27, 34, 35,75] |
Flavonoids | Antioxidant Anti-inflammatory Restore monoamine levels |
[36,79] |
Quercetin | Antioxidant Anti-inflammatory Potentials to interfere with the formation of amyloid-β plaques Stabilises tau protein Potential senolytic Inhibit acetylcholinesterase activity |
[14, 37-39, 63] |
Fisetin | Antioxidant Anti-apoptotic Antiproliferative Neuroprotective Anti-inflammatory Inhibit acetylcholinesterase activity |
[40-42] |
Isothiocyanates | Anti-inflammatory Neuroprotective Inhibit the production of pro-inflammatory cytokines |
[35, 43, 44] |
Tannins | Neuroprotective Anti-inflammatory |
[43, 45, 55] |
Alkaloids | Inhibit acetylcholinesterase activity Anti-inflammatory Antioxidant Anti-amyloidogenic Increase dopaminergic activity |
[46, 47,75] |
Vitamins (C, E, and A) | Antioxidant Maintain cellular function Oxidative damage protection |
[48, 49] |
Amino Acids, Minerals, and Fatty acids | Nutrient contribution | [50] |
2.2. Alkaloids, Glucosinolates and Isothiocyanates
Alkaloids are compounds that contain mostly basic nitrogen atoms. Alkaloids isolated from M. oleifera leaves including N,α-l-rhamnopyranosyl vincosamide, phenylacetonitrile pyrrolemarumine, 4′-hydroxyphenylethanamide-α-l-rhamnopyranoside and its glucopyranosyl derivative [27]. Glucosinolates and isothiocyanates are sulfur-containing compounds found in M. oleifera. Isothiocyanates are bioactive products formed when glucosinolates are hydrolyzed by the enzyme myrosinase. 4-(α-L-rhamnosyloxy) benzyl glucosinolate (glucomoringin) is the predominant glucosinolate found in M. oleifera, with 4-(α-L-rhamnosyloxy) benzyl isothiocyanate (moringin) serving as its corresponding isothiocyanate [30].
2.3. Saponins
M. oleifera is a plentiful source of saponins, with their concentrations differing depending on the plant part and the extraction method used. The leaves typically exhibit higher saponin content compared to seeds [27, 31]. Studies have demonstrated that its leaves contain a substantial amount of saponins, with reported concentrations of approximately 0.95 g per 100 g of leaf extract. Comparative analysis reveals that while saponins are present in M. oleifera seeds, their concentration is notably lower than that of flavonoids and alkaloids. For instance, one study reported saponin levels at approximately 0.06 g per 100 g of seeds [32]. This indicates that although saponins contribute to the phytochemical profile of the seeds, they are not the predominant compounds, as flavonoids and alkaloids are more abundant.
2.4. Tannins
Tannins are water-soluble phenolic compounds known for their ability to precipitate alkaloids, gelatin, and other proteins [27]. Immature M. oleifera leaves are reported to have slightly higher tannin levels, particularly from partially harvested or severely treated trees. However, the concentration of tannins is typically lower than that of flavonoids, polyphenols and saponins in M. oleifera extracts [33].
3. EXTRACTION AND ANALYTICAL TECHNIQUES
Various extraction techniques are utilized to isolate bioactive compounds from different parts of M. oleifera, such as its leaves, pods, and seeds. Commonly used methods include solvent extraction with ethanol, methanol, or water [51], supercritical fluid extraction (SFE) [52], microwave-assisted extraction (MAE) [53], and others. Each method offers distinct advantages in efficiency, selectivity, and environmental impact, depending on the specific phytochemicals being targeted.
Analyzing the bioactive compounds in M. oleifera involves employing a suite of chromatographic and spectroscopic techniques that collectively provide detailed insights into its bioactive constituents. High-Performance Liquid Chromatography (HPLC) is a cornerstone technique for separating and quantifying individual phenolic compounds, flavonoids, and vitamins within M. oleifera extracts. This method offers high sensitivity and specificity, essential for identifying and quantifying bioactive components amidst complex matrices [54]. Meanwhile, Gas Chromatography-Mass Spectrometry (GC-MS) plays a pivotal role in analyzing volatile compounds and lipid-soluble vitamins present in M. oleifera extracts.
This technique provides critical data on the nutritional and medicinal properties of the plant, offering detailed insights into its chemical composition [55]. Additionally, Nuclear Magnetic Resonance (NMR) spectroscopy allows for the analysis of complex samples, such as herbs and phytomedicines, with high accuracy and consistency without the need for separation. It provides a detailed profile of each sample of the molecular structure of bioactive compounds [56]. It is used to confirm the identity of compounds extracted from M. oleifera and to study their interactions with biological targets relevant to AD. The spectroscopic technique, UV-Vis spectrophotometry, is utilized for rapid quantification of total phenolic content, total flavonoid content, and antioxidant activity within M. oleifera extract [57]. These measurements are crucial for assessing the bioactivity and potential health benefits of M. oleifera products.
The standardization of M. oleifera extracts is essential to ensure consistent levels of key bioactive compounds across different batches, thereby enhancing their reliability and efficacy. This rigorous process is essential for maintaining the efficacy and safety of Moringa-based products intended for therapeutic applications, including those aimed at mitigating AD symptoms. Understanding the intricate phytochemical composition of M. oleifera through robust extraction and analytical methodologies is pivotal in unlocking its therapeutic potential, particularly its purported neuroprotective effects. Continued research efforts focused on refining extraction techniques and exploring synergistic interactions among bioactive compounds will further enhance our understanding and harness the full therapeutic benefits of this versatile botanical resource.
4. PHARMACOLOGICAL PROPERTIES RELEVANT TO ALZHEIMER’S DISEASE
M. oleifera, a plant renowned for its rich nutritional profile and bioactive compounds has garnered significant attention for its potential therapeutic effects in AD. The mechanisms by which M. oleifera exerts its beneficial effects in AD are multifaceted and involve several key pathways, which are summarized in Fig. (1).
4.1. Antioxidant
The antioxidant properties of M. oleifera are primarily attributed to its high content of bioactive compounds, including flavonoids, phenolics, and other phytochemicals. These compounds have been shown to scavenge free radicals and enhance the activity of endogenous antioxidant enzymes, thereby reducing oxidative damage in neuronal tissues. For example, studies have demonstrated that Moringa leaf extracts can significantly lower malondialdehyde (MDA) levels, a marker of lipid peroxidation, and increase glutathione (GSH) levels, indicating improved antioxidant status in animal models exposed to oxidative stress [58].
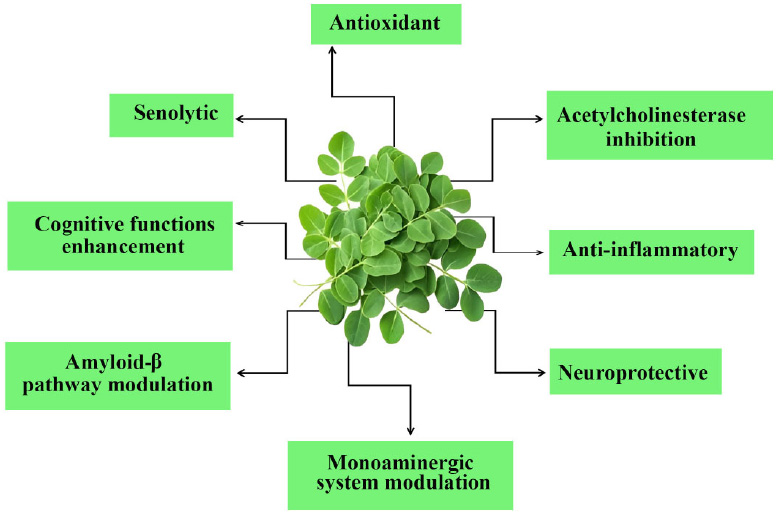
Pharmacological properties of M. oleifera relevant to Alzheimer’s disease.
Furthermore, it has been reported that M. oleifera activates various antioxidant pathways, which contribute to its neuroprotective effects against oxidative stress-induced neuronal damage [59]. Phenolic compounds play a crucial role in exhibiting antioxidant properties [36], with plants containing higher concentrations of polyphenolic compounds typically exhibiting stronger antioxidant activity. M. oleifera has been extensively studied for its antioxidant properties. In 2011, Tesfay et al. conducted a comparison study to investigate the antioxidant content in various parts of M. oleifera [60]. Their findings align with those of Perry et al., who discovered that the leaves of M. oleifera exhibit the highest antioxidant activity [61]. Both studies reveal that M. oleifera leaves are rich in nutritional antioxidant components such as ascorbic acid, phenols, carotenoids, and tocopherols.
4.2. Acetylcholinesterase Inhibition
A well-known therapeutic approach for controlling AD involves inhibiting acetylcholinesterase, as decreased acetylcholine levels are associated with cognitive decline. M. oleifera has been explored for its potential as an anticholinesterase agent, a property that is significant for managing AD. The mechanism of action involves various bioactive compounds that inhibit acetylcholinesterase (AChE), the enzyme that breaks down acetylcholine, a neurotransmitter essential for memory and learning. Numerous phytochemicals in M. oleifera, such as flavonoids, phenolic acids, and alkaloids, have demonstrated anticholinesterase activity. Extracts from M. oleifera leaves significantly inhibit AChE, which raises acetylcholine levels in the synaptic cleft and may enhance cognitive abilities [62]. Specifically, compounds widely present in M. oleifera, such as quercetin and kaempferol, have been shown to be strong AChE inhibitors and contribute to the plant's overall neuroprotective properties [46, 63].
4.3. Anti-inflammatory
M.oleifera exerts anti-inflammatory effects, which are crucial in the context of AD. Chronic inflammation in the brain is linked to the progression of AD, and M. oleifera has been shown to reduce levels of pro-inflammatory cytokines [64]. This anti-inflammatory action can further support cognitive function by preserving the neuronal environment and enhancing synaptic plasticity. M. oleifera supplementation has been shown to ameliorate homocysteine-induced AD-like pathology in rats, which is characterized by oxidative stress and neuroinflammation [35]. Extracts from M. oleifera have been found to suppress the activation of inflammatory mediators in macrophages, potentially reducing neuroinflammation associated with AD. This anti-inflammatory action is believed to be mediated through the inhibition of the NF-κB signaling pathway, which plays a crucial role in the inflammatory response [65].
4.4. Neuroprotective
One of the primary mechanisms by which M. oleifera exerts its neuroprotective effects is through its potent antioxidant activity. Oxidative stress is a significant contributor to neuronal damage in AD, leading to the degeneration of neurons and cognitive decline. By enhancing the endogenous antioxidant defense systems and reducing oxidative damage, M. oleifera helps to preserve neuronal integrity and function [66]. M.oleifera has been found to enhance neurogenesis and synaptic plasticity, which are vital for cognitive function. The presence of bioactive compounds such as isothiocyanates and flavonoids in M. oleifera has been linked to the promotion of neuronal survival and regeneration [63]. These compounds may stimulate the expression of neurotrophic factors, which support the growth and differentiation of neurons, thereby enhancing cognitive function and memory [67]. Natural antioxidants in M. oleifera act as efficient radical scavengers and metal chelators. It has been demonstrated to protect against hydrogen peroxide-induced damage in neuroblastoma cells by lowering reactive oxygen species (ROS) levels and lipid peroxidation, preventing mitochondrial dysfunction, and boosting GSH content and antioxidant enzyme activity [57]. A model of AD involving the infusion of colchicine into the brain of rats was established by Ganguly & Guha in 2008 [68]. In this study, M. oleifera was found to change brain monoamines and electrical patterns, suggesting that it provides protection against AD. Another in vivo study established a model of AD induced by hyperhomocysteinemia in rats [35]. The study provided evidence that M. oleifera decreases oxidative stress caused by homocysteine, alleviating tau hyperphosphorylation and Aβ pathology. Moreover, M. oleifera’s ability to protect against aluminum chloride-induced neurotoxicity further underscores its potential as a therapeutic agent for AD. This neuroprotective effect is attributed to its antioxidant properties, which helps counteract the oxidative stress induced by aluminum exposure, a metal implicated in the pathogenesis of AD [69]. The presence of compounds such as niazimicin, a thiocarbamate glycoside found in M. oleifera seeds, has also been highlighted for its neuroprotective activity, suggesting that various components of the plant may contribute to its overall efficacy against neurodegeneration [58].
4.5. Modulation of β-amyloid Pathway
The accumulation of amyloid-β peptides is a hallmark of Alzheimer's pathology, leading to neurotoxicity and cognitive decline. M. oleifera contains various bioactive compounds that may influence the production, aggregation, and clearance of these peptides, thereby contributing to its neuroprotective properties. One significant mechanism by which M. oleifera may modulate the β-amyloid pathway is through the inhibition of β-secretase (BACE1), the enzyme responsible for the initial cleavage of the amyloid precursor protein (APP) to produce amyloid-β peptides. Certain phytochemicals found in M. oleifera, such as polyphenols and flavonoids, can inhibit BACE1 activity, thereby reducing the production of amyloid-β [70]. By decreasing the levels of amyloid-β, M. oleifera helps to mitigate the formation of amyloid plaques, which are toxic to neurons and contribute to the progression of AD. Additionally, M. oleifera has been shown to enhance the expression of neprilysin, an enzyme that plays a crucial role in the degradation of amyloid-β peptides. Increased neprilysin activity can facilitate the clearance of amyloid-β from the brain, thereby reducing its accumulation and associated neurotoxicity [71]. This dual action of inhibiting BACE1 while enhancing neprilysin activity positions M. oleifera as a promising therapeutic agent for the management of AD.
4.6. Enhancement of Cognitive Function
Neurogenesis is critical for cognitive function and may be aided by M. oleifera. According to Sutalangka et al. [72], the bioactive compounds found in M. oleifera have been associated with the stimulation of neurotrophic factors, which facilitate the growth and differentiation of neurons. This neurogenic effect has the potential to improve cognitive function and mitigate the neurodegenerative mechanisms associated with AD. However, further studies concerning the active substance are still warranted.
4.7. Senolytic Potential
Recent research suggests that M. oleifera may have senolytic properties, offering potential benefits for age-related disorders. The plant’s antioxidant and anti-inflammatory effects are central to its potential in combating cellular senescence, characterized by oxidative stress and chronic inflammation. For example, M. oleifera has been shown to significantly reduce oxidative stress and inflammation [56, 57, 62], both of which are key contributors to aging. Compounds in M. oleifera such as quercetin, have shown promise in modulating cellular pathways related to senescence [73]. Additionally, it may function as a PI3K/Akt pathway inhibitor, promoting the selective elimination of senescent cells. While the PI3K/Akt signaling pathway promotes cell survival and inhibits apoptosis in healthy cells, M. oleifera could potentially increase the vulnerability of senescent cells to apoptosis-inducing signals [74]. This dual mechanism suggests that M. oleifera may help eliminate senescent cells while preserving healthy neurons.
4.8. Modulation of Monoaminergic Systems
M. oleifera bioactive compounds may positively impact AD through their effects on the monoaminergic system. These compounds can inhibit monoamine oxidase (MAO), the enzyme responsible for serotonin degradation, thereby enhancing serotonin levels, a neurotransmitter essential for mood regulation, appetite, and sleep [75]. A few studies have reported the ability of M. oleifera to modulate neurotransmitter levels, supported by the presence of tryptophan, a precursor of serotonin that facilitates its synthesis [76-78]. Additionally, bioactive compounds such as flavonoids, tannins, isothiocyanates, and polyphenols have been shown to restore monoamine levels in the brain, highlighting their potential in addressing neurodegenerative disorders [75,79,80]. Polyphenols, terpenoids, glycosides, and phytosterols present in M. oleifera are also linked to increased dopaminergic activity, which is critical for memory and learning [1,81]. These neuroprotective effects, primarily through neurotransmitter modulation, suggest that M. oleifera holds promise as a therapeutic candidate for managing AD and other neurodegenerative disorders.
5. OMICS EXPLORATION OF M. OLEIFERA
Exploring the therapeutic potential of M. oleifera through various omics approaches, namely as genomic, transcriptomic, proteomic, and metabolomic, provides a comprehensive strategy to understand its complex therapeutic effects for AD. These omics techniques will also be instrumental in studying the various factors that can influence the efficacy of M. oleifera in managing and treating these conditions. In this review, we discuss these omics technologies, which allow researchers to investigate the molecular underpinnings of M. oleifera's potential therapeutic benefits, offering insights into its mechanisms of action and identifying biomarkers for disease progression and treatment efficacy. By leveraging genomics, transcriptomics, proteomics, and metabolomics, we can gain a deeper understanding of how M. oleifera interacts with biological systems, ultimately enhancing our ability to optimize its therapeutic application in AD management.
5.1. Genomics
According to the World Health Organization (WHO), genomics is defined as the study of an organism’s partial or complete set of genetic or epigenetic sequences, which aims to recognize the morphology and function of these sequences and their downstream gene expression. Genomics is different from genetics because genetics focuses mainly on the function and configuration of a single gene, while genomics includes studies of all genes and the relationships between these genes to find out their impact on an organism’s growth and development [82].
The aim of genomics studies in M. oleifera includes uncovering the genes responsible for its bioactive compounds and their potential neuroprotective effects. Recent advances in sequencing technologies have facilitated a thorough analysis of the M. oleifera genome, revealing gene duplications and functional annotations that may enhance its medicinal properties [83]. By understanding the genetic basis of its phytochemical production, researchers can better grasp how specific genes contribute to the synthesis of neuroprotective compounds like isothiocyanates and flavonoids, which are associated with antioxidant and anti-inflammatory benefits [84].
Based on the importance of the genomics study mentioned above, genomics approach on different Moringa species has enabled the identification of key genes of M. oleifera, such as those associated with rapid growth, high protein composition, tolerance to heat and stress. This will enhance our current understanding of M. oleifera and increase awareness of the benefits associated with different Moringa species.
5.2. Transcriptomics
Transcriptomics is a valuable approach in the study of M. oleifera. It involves analyzing the transcriptome, which is the complete set of RNA transcripts produced by the genome under specific conditions. This approach offers insights into gene expression patterns, regulation, and how M. oleifera responds functionally to different environmental or physiological conditions. Transcriptomic analyses also explore how gene expression changes in response to M. oleifera treatment in AD models. By examining differential gene expression, researchers can pinpoint pathways that are activated or suppressed by M. oleifera extracts. Studies have shown that M. oleifera can influence genes related to oxidative stress and inflammation, which are key factors in Alzheimer’s pathogenesis [85]. Additionally, this approach may uncover how M. oleifera affects neurotrophic factors that support neuronal survival and cognitive function. For instance, Moringin, an isothiocyanate extracted from M. oleifera seed, has been reported to increase the expression of genes involved in neuron cortical development, osteogenesis, and adipogenesis [86].
5.3. Proteomics
Proteomics is defined as the large-scale study of proteins, including their structure, physiological role, and function [87] in an organism, tissue, cell or biofluid [88]. Proteomics can be classified into protein expression, structural and functional [89]. It supplements other technologies, namely genomics and transcriptomics in determining the identity of proteins of an organism and recognizing the structure and functions of a protein [90]. Compared to genomics, it is much more powerful and complicated as it provides a detailed presentation of an organism’s structure and function [91]. Proteomic studies focus on identifying and quantifying proteins influenced by M. oleifera treatment. High-throughput proteomics offers insights into protein dynamics related to neuroprotection and cognitive enhancement. For example, assessing changes in proteins involved in synaptic plasticity, neurotransmission, and neuroinflammation following M. oleifera administration in AD animal models can provide valuable information [92]. This data is essential for understanding how M. oleifera may address the pathological processes associated with AD at the protein level.
5.4. Metabolomics
According to Manchester & Anand, metabolomics refers to the comprehensive analysis profiling of metabolites in a biological specimen through the combination of high-throughout analytical chemistry and multivariate data analysis [93]. It utilizes complementary analytical methodologies such as LC-MS/MS (liquid chromatography-mass spectrometry), GC-MS (gas chromatography–mass spectrometry), and NMR (nuclear magnetic resonance), which are capable of identifying and quantifying many known or unknown metabolites simultaneously [94]. Combined use of multiple analytical approaches as mentioned above, is essential to increase the coverage of metabolites detection that is unable to be attained by a single analytical approach because of the complexity of metabolome and its diverse properties [95]. Metabolomics study is rather challenging when compared to genomics and proteomics as metabolomics targets the measurement of diversified molecules ranging from very water-soluble organic acids to very non-polar lipids [96].
Metabolomics provides a comprehensive analysis of metabolites in biological samples, revealing metabolic changes induced by M. oleifera. This approach can identify specific metabolites altered by M. oleifera treatment, which may act as biomarkers for cognitive function and neuroprotection [97]. For instance, evaluating changes in metabolites related to energy metabolism, neurotransmitter synthesis, and oxidative stress can help elucidate how M. oleifera affects metabolic pathways relevant to AD [98].
6. TOXICOLOGY AND SAFETY
The safety and toxicity of M. oleifera have been evaluated through various studies involving both animal models and human subjects. Based on available evidence, most of these studies have shown positive findings in both animal models and human subjects. These results suggest that M. oleifera is safe for consumption, though further research is needed to confirm its long-term safety. Table 2 summarizes research findings on the safety and toxicity of M. oleifera from several studies conducted in animals and humans.
7. CHALLENGES IN STANDARDIZING M. OLEIFERA
Alzheimer’s disease has long been a significant challenge, affecting individuals and families worldwide for centuries. While more research was done with the aim of improving the condition of AD, the usage of natural chemical compounds from herbs like M. oleifera in the treatment of the disease started to gain more attention among researchers in the past two decades. Despite the growing interest, research on the various mechanisms of action of M. oleifera and its effectiveness in treating AD remains limited. Further investigations are needed to identify the most effective parts of M. oleifera, optimize extraction methods, and determine the most beneficial growth stages to harvest the M. oleifera to enhance its therapeutic properties. The main current challenges faced in standardizing M. oleifera include methods of cultivation, extraction, harvest and studies in its formulation and bioavailability, as illustrated in Fig. (2). Addressing these challenges is crucial for advancing precision medicine approaches in AD treatment, ensuring consistent efficacy and safety across therapeutic applications.
As more beneficial effects of M. oleifera have been discovered, more cultivation of the plant is done worldwide. Growing M. oleifera is not exactly difficult as they can grow in the versatile climates of the tropic and subtropic regions and in a variety of soils, except for waterlogged conditions [114]. However, further studies are needed to determine the optimal cultivation methods and conditions for enhancing both the quality and quantity of M. oleifera products. The cultivation of growing M. oleifera can be achieved through sowing and cutting. The sowing method requires selection and proper storage of seeds as seed germination can decrease when the seeds are kept at ambient temperature with high humidity. Growing from cuttings is another alternative method when there are limited seeds available, but this requires more labour work. The cuttings can readily grow roots and are able to grow into trees within months. When comparing both growing methods, trees that grow from seeds tend to produce a lower quality of fruits but grow longer roots than the cuttings, which provide them with better stabilization and access to water [115]. Thus, the choice of planting method can vary depending on the environmental conditions and the expected yield for different plantations.
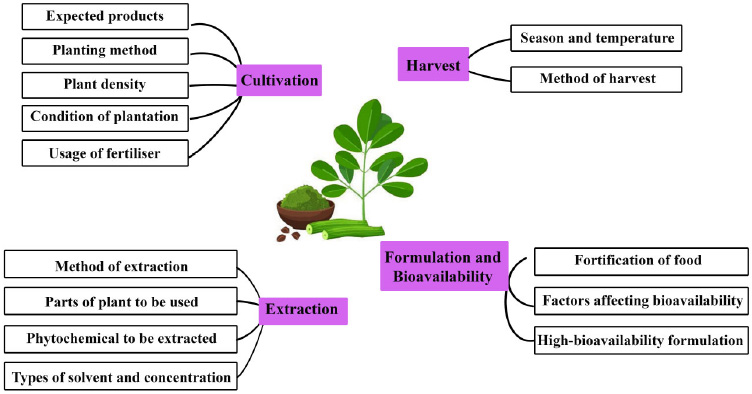
Challenges in standardizing M. oleifera.
Study Type | Experiment Model | Dosage | Findings | References |
---|---|---|---|---|
Animal | Mice | 15.9 and 17.8 g/kg | Induce acute toxicity | [77] |
Mice | 250, 500, and 750 mg/kg | Induce acute and sub-acute liver and kidney toxicity | [99] | |
Mice | 2500 mg/kg | No acute toxicity observed | [100] | |
Rats | 100, 200, and 400 mg/kg | No harmful consequences on the brain | [72] | |
Rats | 250 mg/kg for 14 days | No significant lesions on the liver, kidney, and testes | [101] | |
Rats | 400 mg/kg | No notable toxicity | [102] | |
Rats | 2000 mg/kg | No significant effects were observed on haematological, biochemical parameters and sperm quality. | [103] | |
Rats | 1.5,3.0, 6.0 g/kg | No significant effects on body weight, food intake, or blood index | [104] | |
Albino Rats | 150 mg/ kg | No adverse reaction in the brain | [105] | |
Wistar Rats | 250 mg/kg | No adverse effects on kidney and liver | [106] | |
Wistar Rats | 500, 1000, and 2000 mg/kg | No significant harm to the brain, liver, or kidneys at moderate to low doses | [107] | |
Sprague-Dawley Rats | 1000 and 3000 mg/kg | No death was reported at the highest dose | [108] | |
Sprague-Dawley Rats | 0-1000 mg/kg and 5000 mg/kg | No significant toxic effects were observed at lower doses, and no visible abnormalities in the tubules and glomeruli. | [109] | |
Broiler Chickens | 500 and 1000 mg/kg for 42 days | No adverse effect | [110] | |
Human | 27 participants | 20 g/kg | No adverse effect | [111] |
30 participants | 7g/day for 90 days | No notable toxicity | [77] | |
55 participants | 8g/day for 40 days | No notable toxicity | [112] | |
82 participants | 100 g/week 3 times/day |
No adverse effects on iron status | [113] |
Another aspect to take into consideration is the density of M. oleifera plants, which has a significant impact on their biomass production. Generally, studies show that the higher the plant density (less spacing between the plants), the higher their biomass production. Zheng et al. discovered that an increase in plant density can lead to a decrease in biomass yield per plant, as higher density results in greater sunlight interception, which negatively affects photosynthesis [116]. However, in cases of increased plant density, the decrease in yield per plant can be offset by the larger number of plants, ultimately resulting in an overall increase in total yield. The plantation done by Němec et al. showed a border-effect, where the M. oleifera plants by the border of the plantations appeared to be visibly larger and yielded higher biomass production [117]. Their study also proved that lower plant density leads to lower biomass production. Still, the large spacing between the plants can possibly reduce pests and diseases in the trees due to better air circulation. The study done by Mendieta-Araica et al. shows no significant changes in the nutritional value of M. oleifera with increased plant density [118]. On the other hand, the studies done by Zheng et al. and Mabapa et al. showed significant changes in the plants’ nutrient content with different densities. These differences may be linked to the rainy season and regional temperatures, where higher temperatures and increased rainfall were found to enhance the plant's nutritional value [116, 119]. It is very difficult to specifically determine an optimum plant density that gives the highest yield because it can be affected by other factors such as the soil, temperature, seasons, availability of seeds, pests, diseases and so forth. For future M. oleifera cultivation, it is recommended to use higher plant densities to maximize leaf biomass production, lower densities to optimize seed yield, and intermediate densities to strike a balance between both leaf and seed production [105].
The harvesting of M. oleifera is also important in ensuring a better quality of yield and maintaining the condition of the plant. Maximum fresh biomass can be harvested during hot and rainy seasons [119]. The harvest can be performed either mechanically or manually by directly plucking them off the plant. Despite being a quicker method, manually plucking from the plant can result in a slower regrowth of the plant [115]. Besides, the usage of fertilizer can enhance the growth of the shoots and roots of the plants as they increase the availability of nutrients like nitrogen and phosphorus in the soil. The organic matter in the fertilizer can also reduce the toxic effects of iron and aluminium in the soil, thereby reducing the root toxicity caused by high levels of aluminium [120, 121]. In a prolonged period of cultivation, there can be great nutrient depletion from the soil, causing decreased yield from the plants. Fertilizers can be used to maintain the nutrients of the soil and ensure a good yield [118]. Hence, harvests of M. oleifera are preferred to be done during rainy seasons and at higher temperatures by using tools like a machete. The usage of fertilizer can also be considered to increase the yield of the plants, but this should be done wisely by choosing a suitable fertilizer that is relatively safe for humans.
Extraction methods are important to liberate maximum active compounds from the M. oleifera plant. According to the study by Ahmed et al., the phytochemicals found in medical plants that showed the highest AChE inhibition property are the alkaloids, followed by terpenes, sterols, flavonoids, and glycosides [122]. These phytochemicals can also be seen in M. oleifera plants. Various extraction methods have been employed at different temperatures using different types and concentrations of solvents, including techniques like maceration, shaking, stirring, ultrasonic extraction as well as cold and hot extractions. Different studies were also done to extract phytochemicals from various parts of the plant, including the leaves, flowers, seeds, stems, barks and roots [22]. Despite several studies regarding the extraction of phytochemicals from M. oleifera and the preferred methods and conditions for extraction of each specific compound, there remains a strong need for further extraction experiments. This is because the results may vary depending on the origin and growing conditions of the plants. The results may also possibly be affected by the methods of handling the experiments by different experimenters. Conducting more experiments will allow for comparisons between different conditions, leading to more accurate and reliable data.
To date, various parts of M. oleifera are consumed by many people due to their nutritional value. M. oleifera can also be incorporated into other foods to enhance their nutritional profile. Giuberti et al. found that adding M. oleifera leaf and seed powder into cereal-based food increased their protein, dietary fiber, and mineral content [123]. However, the fortification of food with M. oleifera has yet to gain widespread acceptance, as it can alter the taste and appearance of the food. Nambiar & Seshadri investigated the bioavailability of β-carotene from both fresh and dried M. oleifera leaves, either finely minced or powdered, when added to food in rat models. Their findings indicated that both preparation methods resulted in high bioavailability of β-carotene in the rats [124]. Another study by Saini et al. in rat models showed approximately 82% bioavailability of folate compared to an equivalent amount of folic acid [125]. An interesting study by Dai et al. showed an increased calcium bioavailability of M. oleifera leaves in rat models after fermenting the leaves with lactic acid bacteria and yeasts [126]. Research has shown that M. oleifera has positive AChE inhibiting activity [61]; however, there is a notable gap in studies aimed at formulating M. oleifera into a highly bioavailable remedy for AChE inhibition as a treatment for AD. Factors that may affect their bioavailability, including absorption, metabolism, and preparation methods, should be carefully considered. Other alternatives, like the incorporation of M. oleifera into foods, may also be explored for neuroprotective purposes in supplement formulations.
CONCLUSION
M. oleifera holds promising potential as a therapeutic approach for AD, underpinned by its rich an array of bioactive compounds that contribute to various pharmacological properties, including antioxidant, acetylcholinesterase inhibition, anti-inflammatory effects, neuroprotection, modulation of the β-amyloid pathway, enhancement of cognitive function, senolytic potential and modulation of monoaminergic systems. The incorporation of various omics technologies presents a cutting-edge approach to expand upon our understanding of the molecular mechanism and therapeutic advantages of M. oleifera, paving the way to novel approaches in the management of AD. However, the challenges in standardizing M. oleifera, such as variations in extraction techniques, plant growth conditions, and formulation strategies, need to be systematically addressed to optimize its clinical efficacy and safety. While the safety profile of M. oleifera is generally favorable, further research is essential to assess potential toxicity and determine optimal therapeutic dosages. In conclusion, the treatment potential of M. oleifera in AD is multifaceted. Future treatments may involve combining M. oleifera’s bioactive compounds with existing therapies to target multiple pathways, including neuroinflammation, oxidative stress, and neuronal dysfunction. Specific treatment avenues include the use of M. oleifera as a supplement for enhancing cognitive function, as an anti-inflammatory agent to reduce neuroinflammation, and as a senolytic to target senescent cells in the brain. Additionally, its role in regulating neurotransmitter levels and modulating the β-amyloid pathway could provide complementary benefits to current pharmacological approaches. To establish M. oleifera as a viable therapeutic option, rigorous clinical trials, standardized formulations, and further exploration of its therapeutic dosages are needed. By overcoming these challenges, M. oleifera could emerge as a valuable therapeutic option in AD management, ultimately contributing to enhanced cognitive health and quality of life for affected individuals and their caretakers.
AUTHORS’ CONTRIBUTION
It is hereby acknowledged that all authors have accepted responsibility for the manuscript's content and consented to its submission. They have meticulously reviewed all results and unanimously approved the final version of the manuscript.
LIST OF ABBREVIATIONS
SFE | = Supercritical fluid extraction |
MAE | = Microwave-assisted extraction |
APP | = Amyloid precursor protein |
Aβ | = Amyloid beta peptide |
WHO | = World Health Organization |
AD | = Alzheimer’s disease |
FUNDING
This work was supported by the Ministry of Education Malaysia through Fundamental Research Grant Scheme (FRGS/1/2024/SKK10/MUCM/02/1), MUCM-MRB joint seed grant (MUCM-MRB/001/2024) and Manipal University College Malaysia Research Grant Scheme 2022 (MUCM/001/2022).
ACKNOWLEDGEMENTS
Declared none.