All published articles of this journal are available on ScienceDirect.
Urolithins–gut Microbial Metabolites with Potential Health Benefits
Abstract
Urolithins are bioactive metabolites derived from the gut microbial transformation of ellagitannins found in various foods like pomegranates, berries, and nuts. These metabolites, primarily urolithin A and urolithin B, exhibit diverse biological activities, including anti-inflammatory, antioxidant, anticancer, neuroprotective, antiatherogenic, antidiabetic, and anti-obesity effects. Their mechanisms of action involve modulating various signaling pathways, reducing oxidative stress, and promoting beneficial gut microbiota. Urolithins have been shown to protect cells from oxidative stress, reduce inflammation, and inhibit tumor growth. They also have neuroprotective effects, potentially mitigating neurodegenerative diseases like Alzheimer's and Parkinson's. Additionally, urolithins may contribute to cardiovascular health by reducing cholesterol levels and improving endothelial function. However, the bioavailability and production of urolithins can vary significantly among individuals due to differences in gut microbiota composition. To enhance their therapeutic potential, researchers are investigating strategies to improve bioavailability and optimize production. While preclinical studies have demonstrated the promising effects of urolithins, further clinical research is necessary to fully understand their efficacy and safety in humans. By addressing the challenges associated with bioavailability and individual variability, urolithins may offer a novel therapeutic approach for a variety of diseases. This review article aims to synthesize recent literature on urolithins, with a focus on their potential health benefits, bioavailability, and applications in disease prevention and treatment.
1. INTRODUCTION
A literature search was conducted in the PubMed database using keywords and word combinations related to the research topic. The search was performed in specific categories (clinical studies, systematic reviews, abstract reports, basic research studies), and was limited to articles published mostly in the last five years. MeSH terms (Medical Subject Headings) from PubMed ensured that the search covered all relevant topics, regardless of the use of different words in the title or abstract.
Urolithins are gut-derived metabolites produced through the microbial degradation of ellagitannins, which are polyphenolic compounds naturally found in fruits, nuts, and oak-aged products. Among these metabolites, Urolithin A (UA) and Urolithin B (UB) have demonstrated significant biological activities, including antioxidant, anti-inflammatory, anti-aging, and anticancer effects [1]. Additional derivatives, such as Urolithin M-5, Urolithin M-6, Urolithin M-7, Urolithin C, and Urolithin E, serve as intermediates in the conversion process leading to UA formation (Fig. 1) [2]. However, the bioavailability of urolithins is highly variable due to differences in gut microbiota composition, which influences their metabolic transformation [3]. To address this limitation, innovative delivery approaches, such as liposomal encapsulation and nanoparticle-based systems, have been developed to enhance absorption and therapeutic potential [4]. Scientific interest in urolithins continues to grow due to their promising role in preventing chronic diseases, including cancer, neurodegenerative disorders, and cardiovascular conditions. This review explores recent advances in urolithin metabolism, bioavailability, health benefits, and therapeutic applications.
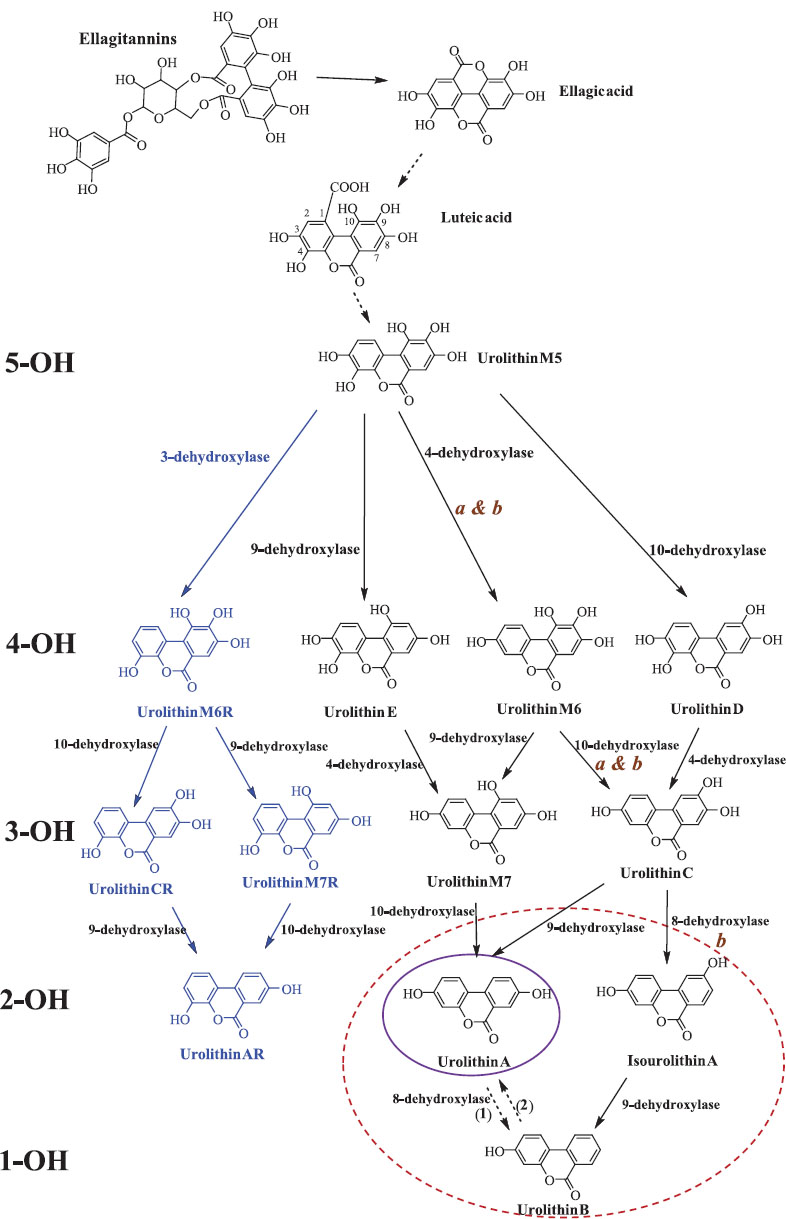
Catabolic pathway of ellagitannins and ellagic acid to urolithins. 5-OH, OH, and 1-OH refer to the number of hydroxyl groups for each urolithin group—penta-, di- and monohydroxy urolithins, respectively. Note: The blue font refers to new urolithins a bacterial 3-dehydroxylase. The purple and red circles designate the final urolithins UM-A and UM-B, respectively. Uro-AR can be found in both metabotypes [2].
2. METABOLISM AND BIOAVAILABILITY OF UROLITHINS
The specific type of urolithins formed depends on the unique bacteria present in the gut. These metabolites undergo further modifications in the large intestine and liver, influencing their ability to circulate in the bloodstream and reach target tissues. However, their bioavailability is limited by several factors, including low water solubility and inter-individual differences in gut microbiota composition. It is estimated that only 40% of individuals can effectively convert polyphenolic precursors into UA. Three primary metabotypes have been identified:
• Metabotype A: Produces only UA.
• Metabotype B: Produces UA, UB, and isourolithin A.
• Metabotype 0: Does not produce detectable urolithins [5].
2.1. Encapsulation Strategies and Mechanisms
Due to poor water solubility and susceptibility to enzymatic degradation, urolithin bioavailability remains a challenge. Encapsulation strategies, such as liposomal and nanoparticle-based delivery, have shown promise in improving stability and absorption. These systems protect urolithins from degradation and facilitate better intestinal absorption.
Liposomal encapsulation involves embedding UA in phospholipid bilayer vesicles, enhancing its stability and absorption. Hu et al. (2023) demonstrated that pH-driven liposomal encapsulation significantly improved UA stability, bioaccessibility, and bioavailability [6]. Compared to non-encapsulated UA, liposomal formulations resulted in significantly higher intestinal absorption and plasma concentrations. PEGylated liposomes further prolonged circulation time and enhanced therapeutic efficacy [7].
Nanoparticles, including Solid Lipid Nanoparticles (SLNs), have been investigated as carriers for UA. Al-Fatlawi et al. (2024) developed SLNs loaded with methylated UA, yielding monodisperse nanoparticles (~84.8 nm) with high drug-loading efficiency (88.6%) [8]. These nanoparticles demonstrated selective cytotoxicity against cancer cells, improved cellular uptake, and enhanced bioavailability compared to free UA. Additionally, lipid nanoparticle-based formulations exhibited superior stability and protection against gastrointestinal degradation [9]. Both liposomal and nanoparticle-based delivery systems enhance gut absorption by shielding UA from enzymatic degradation and facilitating transport across the intestinal epithelium. Studies have shown that nanoparticle formulations achieve higher plasma concentrations and improved therapeutic outcomes. Lin et al. reported that solid lipid nanoparticles increased the oral bioavailability of bioactive compounds by optimizing lymphatic transport and cellular uptake mechanisms [10]. Improvement in oral bioavailability of UA and protection from side effects of cis-platin anticancer drugs in mouse models was achieved by formulating it into biodegradable nanoparticles that use a surface-conjugated ligand targeting the gut-expressed transferrin receptor. Nanoparticle encapsulation of UA led to a sevenfold enhancement in oral bioavailability compared with native UA [11].
3. HEALTH BENEFITS OF UROLITHINS
3.1. Anti-inflammatory Effects
Urolithins, particularly Urolithin A, have shown potent anti-inflammatory effects, which are crucial in managing chronic inflammatory diseases [12]. Urolithins suppress the production of pro-inflammatory cytokines such as TNF-α and IL-6 [1]. The modulation of these cytokines plays a critical role in diseases like rheumatoid arthritis, inflammatory bowel disease, and neuroinflammation.
In addition, studies suggest that Urolithin A can reduce inflammation by inhibiting NF-κB signaling pathways, which are key regulators of the inflammatory response. This makes urolithins promising candidates for treating inflammatory conditions. More specifically, the possible mechanisms of the anti-inflammatory action of UA and UB in LPS-treated BV2 mouse microglia are through inhibition of NF-B p65, Erk1/2, p38 MAPK, and Akt phosphorylation and signaling pathways. The two urolithins suppressed the mRNA levels of proinflammatory TNF-α, IL-6, IL-1, iNOS, and COX-2 [1]. Urolithin B was shown to reduce the levels of IL-1β, IL-6, and TNF-α in the brain of a D-galactose-induced mouse model of Alzheimer's disease. It also decreased the phosphorylation of ERK, p38, AKT, and NF-κB, indicating its potential anti-neuroinflammatory effects [13]. In RAW264 macrophages, Urolithin A was found to suppress the production of pro-inflammatory mediators NF-κB and AP-1 by modulating the PI3K/Akt/NF-κB and JNK/AP-1 signaling pathways in experimental animals [3, 14].
3.2. Antioxidant Properties
Oxidative stress illustrates, in a way, the imbalance between “creation” and “destruction” of free radicals. Satisfactory levels of antioxidants can cope with short periods of oxidative stress, while prolonged periods of oxidative stress deplete the body's reserves of antioxidants. The “Reactive Oxygen Species” (ROS) are classified into the following four categories: (i) free radicals, such as the hydroxyl radical (∙OH) (ii) ions, such as the hypochlorite anion (ClO-), resulting from the dissociation of hypochlorous acid (HClO) (iii) combinations of free radicals and ions, such as the superoxide anion (∙O2-) and (iv) molecules, such as hydrogen peroxide (H2O2). ROS causes peroxidative damage to lipids, proteins, and DNA, leading to cancer, premature aging, and degenerative diseases [15]. The effects of oxidative stress depend on the extent of these changes: The cell can recover its original state after limited oxidative damage. More severe perturbations, however, lead to cell death, either by the process of apoptosis (when moderate) or by direct cell necrosis (when very strong).
The antioxidant potential of urolithins is another area of growing interest. Urolithins scavenge free radicals and enhance the activity of endogenous antioxidant enzymes like Superoxide Dismutase (SOD) and catalase. These effects help in mitigating oxidative stress, which is implicated in the pathogenesis of numerous chronic diseases, such as cancer, cardiovascular diseases, and neurodegenerative disorders. In the human neuroblastoma cell line SK-N-MC, UA significantly reduced ROS production and oxidative stress-induced apoptosis, increased cell viability, reduced Bax/Bcl-2 ratio, suppressed phosphorylation of the p38MAPK pathway, and improved mitochondrial function through the oxidative stress-associated SIRT1/PGC-1 pathway [1]. Also, Uro-A can inhibit the apoptosis of cells involved in oxidative stress by reducing ROS production in cells, inhibiting the mitochondrial apoptosis pathway, and modulating the p-38 MAPK pathway [3, 16, 17].
3.3. Anticancer Activity
One of the most studied aspects of urolithins is their anticancer activity. Urolithin A and Urolithin B have shown the ability to induce apoptosis, inhibit cell proliferation, and arrest the cell cycle at various stages. These effects are especially pronounced in prostate, colon, and breast cancer cells [11, 18]. Urolithin A has been shown to modulate key signaling pathways, such as PI3K/Akt and Wnt/β-catenin, which are involved in cancer cell survival and proliferation. The PI3K/AKT/mTOR signaling pathway is considered to be the central regulatory pathway in translation, regulating processes such as cell growth and division, differentiation, and cell survival. New data have shown that increased AKT/mTOR activation leads to tumorigenesis, whereas the inhibitory effect of urolithins on cardiomyocyte apoptosis is mediated in part through activation of PI3K/Akt signaling [19]. Moreover, Urolithins have demonstrated chemo-preventive properties by enhancing the body's ability to detoxify carcinogens through the modulation of phase II detoxifying enzymes. These findings indicate that urolithins could be beneficial in both cancer prevention and therapy.
In colon cancer cell lines, Uro B exerts antitumor activity mainly through the promotion of apoptosis, activation of effector caspase 3, and cell cycle arrest [13, 20]. According to the literature, Uro B has anticancer properties activity, particularly in hepatocellular carcinoma. In vitro study showed that Uro B inhibits the growth of HepG2 cells by increasing the expression of cell cycle proteins and apoptosis regulatory molecules, such as p53 and p38-MAPK, and through enhancing the expression of phosphorylated β-catenin. β-Catenin is a dual-function protein involved in the regulation and coordination of cell-cell adhesion and gene transcription [21, 22]. TP53 is a target for Uro-A to exert antitumor activity. The tumor suppressor gene TP53, also known as “guardian of the genome,” encodes a transcription factor that plays an important role in DNA repair, cell cycle regulation, and apoptosis, and is found mutated in over half of all cancers [23]. Therefore, Uro-A works by activating TP53 [3]. Urolithins have also been found to lower the oxidative stress state in colorectal cancer by reducing intracellular ROS and malondialdehyde and increasing SOD activity in H2O2-treated Caco-2 cells (from Cancer coli, “colon cancer”) [14]. Besides, the antioxidant and anti-inflammatory properties of UA could stimulate tumor growth in parallel with Cisplatin, one of the first-line cytotoxic drugs, eliminating the produced acute kidney injury [9, 24].
3.4. Neuroprotective Effects
Recent studies have also suggested that urolithins may exert neuroprotective effects. Urolithin A has shown potential in protecting neuronal cells against oxidative damage and apoptosis. These properties make urolithins attractive candidates for preventing or managing neurodegenerative diseases such as Alzheimer's disease and Parkinson's disease. The neuroprotective mechanisms of urolithins involve the activation of autophagy and the reduction of mitochondrial dysfunction, which are critical in maintaining neuronal health [25].
The Monoamine Oxidase (MAO) enzymes (MAO-A and MAO-B) are responsible for the inactivation of neurotransmitters and have been linked to depression and Parkinson's disease. Treatment with UA, UB, and UC suppressed MAO-A activity, but none of the tested urolithins showed strong inhibitory activity against MAO-B. Similarly, UA inhibited MAO-A and tyrosinase, acted as a free radical scavenger, and improved the physiological antioxidant defense system in Neuro-2a cells, and the APP/PS1 mice model [1, 3, 13, 22]. The neuroprotective effects of Uros appear to be exerted through their interaction with the gut microbiota. Low microbial diversity, reduced production of butyric acid-producing bacteria, and increased production of enterobacteria, increase intestinal permeability and activate the production of pro-inflammatory cytokines that can cross the blood-brain barrier, resulting in neuroinflammation. Gordonibacter bacterium, which produces urolithins, is considered an inflammatory bacterium that inhibits T helper cells (TH17) [17, 20, 25-28].
3.5. Antiatherogenic Effects
Atherosclerosis is a common damage that is progressively created when the walls of the arteries and vessels of the body's circulatory system begin to suffer damage. These lesions slowly cause the formation of atheromatous plaques on the inner side, mainly of the arteries, something like a form of extensive aggregates that are composed of fatty compounds. Urolithins, as mixtures in a concentration, such as those ingested through ellagitannins in fresh raspberries or raspberry juice, may influence key processes in the development and evolution of atherosclerosis and cardioprotective activity. Uro A and Uro B at 10 mM significantly reduce cholesterol accumulation. Uro C can significantly reduce cholesterol accumulation in macrophages in the culture of Human Umbilical Vein Endothelial Cells (HUVECs). The uptake of LDL by macrophages is via the LDL receptor (LDLr) or through macroporinocytosis, which has been shown to induce foam cell formation, and is a novel target for reducing cholesterol accumulation in the atherosclerotic plaque [29-32].
Once absorbed, urolithins A and B can protect against various diabetes complications. In mice, urolithin A lowers blood sugar, improves insulin response, protects pancreatic cells, and reduces oxidative stress. Urolithin B reduces fat buildup in blood vessels, promotes cholesterol removal, and protects cardiac function in diabetic rats. Both urolithins reduce body weight and fat mass, lower cholesterol levels, and protect cells from inflammation and oxidative stress [20, 33].
In diabetic patients, the effect of UA on Akt phosphorylation (pAkt) in endothelial cells was among the strongest inhibitors of pAkt, suggesting its potential as a therapeutic candidate agent against diabetic podocytopathy by reducing ROS levels [1, 34]. In an E-deficient mouse model (apoE−/−), administration of 10 mg/kg Uro B showed protective and therapeutic effects against atherosclerosis, and Uro B lowered lipid plaque deposition inhibiting lipid uptake and cholesterol efflux in ox-LDL treated in murine J774 and human THP-1macrophage cell lines [3, 13].
Preclinical experiments in rodent models show that polyphenols may have antidiabetic effects dependent on their absorption rate or through the regulation of gut microflora [18].
Renal Ischemia Reperfusion Injury (IRI) is an acute kidney injury associated with a high mortality rate. Transcription factor EB is a protein that is encoded by the TFEB gene in humans. Overexpression of TFEB in cultured cells induces lysosomal biogenesis, exocytosis, and autophagy. Pretreatment with urolithin A promoted autophagy, and showed an inverse relationship between the TFEB-CLEAR pathway and renal injury [35].
3.6. Immune System/microbiota Effects
The human microbiota acts as a natural barrier to protect the host from external pathogens by producing antimicrobial compounds and participating in competitive exclusion. Prevotella, Ruminococcus, Bacteroides, and Firmicutes are some of the major bacterial families that can be found in the human gut. The anaerobic bacteria Peptostreptococcus, Bifidobacterium, Lactobacillus, and Clostridium can be found in the large intestine, which has low oxygen levels. It is believed that these microbes, which compete for nutrition and places to attach to the intestinal mucosa, a key site of immune activity and antimicrobial protein synthesis, inhibit the expansion of dangerous bacteria. The microflora plays a key role in the host's immune system and in the development of the intestinal mucosa. Many diseases, including infections, liver disorders, gastrointestinal cancers, metabolic disorders, respiratory diseases, mental or psychological illnesses, and autoimmune diseases, have been linked to microbial symbiosis.
Polyphenols act by inhibiting the process of lymphocyte differentiation and interleukin secretion, partly determining the immune response. They also inhibit COX-2 expression, Prostaglandin E2 (PGE2) activation, and STAT1 binding to the Interferon Regulatory Factor 1 (IRF-1) promoter in response to IFNγ. Epidemiological and clinical studies highlight the important relationship between gut microbiota, colon inflammation, and colon cancer, while inflammation is enhanced by high-fat diet/ low-fiber diets, family history, and abnormal gut microbial composition [18].
3.7. Anti-obesity Effects
Uro-A promotes heat production in brown adipose tissue, increases energy expenditure, inhibits the enzyme activity of lipase, α-glucosidase and dipeptidyl peptidase-4 in 3T3-L1 adipocytes, and significantly reduces triglyceride accumulation and expression of adipogenesis-related genes. In 3T3-L1 white fat cells, Uro-A increases the expression of brown fat-specific genes by activating the β3-AR-p38 MAPK signaling pathway [3].
3.8. Anti-aging Effects
Uro-A can increase the expression of type I collagen and inhibit metalloprotease-1. At a concentration of 50 µM, it can block the G2/M phase of the cell cycle, achieving an anti-aging effect [36]. In neurodegenerative diseases caused by aging, Uro-A prevents brain aging by activating the SIRT1/mTOR signaling pathway in experimental animals [37]. Table 1 summarizes the health benefits of urolithins, mechanisms of action, and supporting studies.
4. UROLITHINS AND METABOTYPES PRE-CLINICAL AND CLINICAL STUDIES
Although, the metabolism of Ellagic Acid (EA) by gut microbiota leads to the production of urolithins, there is considerable variability between individuals regarding the specific types and quantities of urolithins generated. In other words, different people do not produce the same urolithins or in equal amounts when exposed to Ellagitannins (ET) or EA. Since, urolithin synthesis is primarily influenced by gut microbiota, the unique microbial composition of each person plays a crucial role in determining the presence and availability of various urolithins [38]. The ability to convert EA into different urolithins is categorized into metabotypes, a classification that has evolved over time. The most recent system divides individuals into three groups: Metabotype A (MetA), which produces urolithin A and its conjugates; Metabotype B (MetB), which generates urolithin B, isourolithin A, and urolithin A; and Metabotype 0 (Met0), which does not produce detectable levels of any urolithins (Fig. 2) [39, 40].
Additionally, the interaction between (poly)phenols and gut microbiota is bidirectional—while the microbiota influences metabolite production, (poly)phenol consumption also affects the microbial population. Consequently, some individuals classified as Met0 may transition to MetA or MetB following prolonged or high exposure to ETs or EA [39, 40]. The prevalence of different metabotypes appears to be influenced by various factors. A study of a large clinical study found that metabotype distribution changes with age, with a decline in MetA prevalence, and an increase in MetB until around 30–40 years old, after which the ratio stabilizes. However, approximately 10% of individuals remain Met0 regardless of age [5].
Stratifying participants by metabotype in dietary intervention studies could help reduce variability in research findings related to (poly)phenol consumption effects. There is also growing interest in investigating the relationship between metabotype, individual characteristics, and disease risk. One study suggested that the prevalence of MetB increases in individuals with conditions linked to gut dysbiosis, such as metabolic syndrome or colorectal cancer, though this finding was confirmed only in adults, and did not hold when broader age groups were considered [39, 40]. Additionally, no association was found between metabotype and gender, medication use, or adherence to the Mediterranean diet [5]. Another study comparing individuals with normal weight, overweight-obesity, and metabolic syndrome found that obese individuals capable of producing urolithin A, isourolithin A, and/or urolithin B had a higher cardiovascular risk — based on lipid profiles and plasma glucose— than obese individuals who exclusively produced urolithin A [41].
Urolithin metabotypes serve as potential biomarkers for cardiometabolic health, impacting conditions such as obesity, diabetes, and Cardiovascular Disease (CVD). A study on overweight and obese adults supplemented with 500 mg or 1,000 mg of Urolithin A for 28 days showed significant improvements in mitochondrial function, reduced inflammatory markers (IL-6, TNF-α), and enhanced muscle endurance [42]. Individuals with metabotype A had greater reductions in oxidative stress markers and lipid peroxidation, suggesting a cardioprotective role of UA compared to metabotypes B and 0 [41].
Clinical trials on pre-diabetic individuals have shown that UA supplementation improves insulin sensitivity and glucose metabolism by enhancing mitochondrial biogenesis in skeletal muscle [33]. In another Randomized Controlled Trial (RCT) on Alzheimer's patients, UA administration was associated with increased Brain-Derived Neurotrophic Factor (BDNF) levels, reduced neuroinflammation, and improved cognitive performance [20].
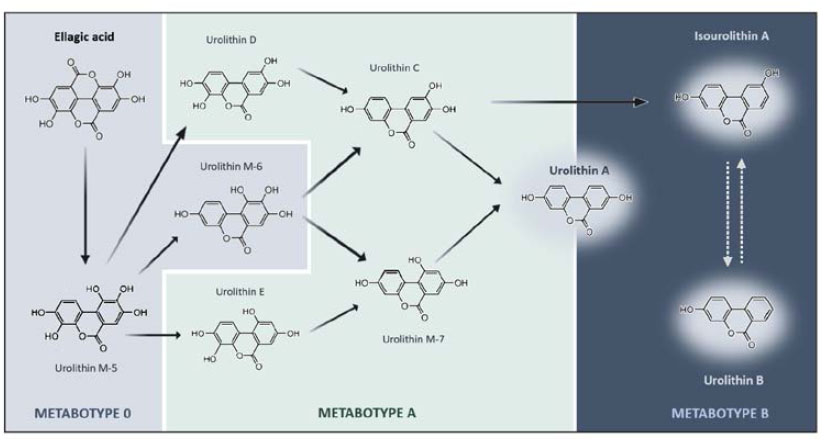
Schematic representation of ellagic acid catabolic pathway by colonic microbiota and the respective urolithin metabotypes [33].
Health Benefit | Mechanism of Action | Supporting Studies/Refs. |
---|---|---|
Anti-inflammatory Effects | Suppresses pro-inflammatory cytokines (TNF-α, IL-6, IL-1β); inhibits NF-κB, PI3-K/Akt, and MAPK pathways; reduces neuroinflammation in Alzheimer’s models. | [1, 12-14, 24] |
Antioxidant Properties | Scavenges free radicals (ROS); enhances SOD and catalase activity; inhibits oxidative stress-induced apoptosis; modulates SIRT1/PGC-1 pathway. | [14, 15, 17, 24] |
Anticancer Activity | Induces apoptosis; inhibits PI3K/Akt and Wnt/β-catenin pathways; activates TP53; modulates β-catenin signaling; reduces ROS in colorectal cancer cells. | [11, 13, 14, 18-24] |
Neuroprotective Effects | Inhibits MAO-A; activates autophagy; reduces mitochondrial dysfunction; improves gut microbiota diversity to reduce neuroinflammation. | [1, 13, 18, 20, 29-34] |
Antiatherogenic Effects | Lowers cholesterol accumulation; inhibits LDL uptake by macrophages; reduces lipid plaque deposition; improves insulin response in diabetic models. | [1, 2, 13, 17, 20, 22, 25, 28, 43, 45] |
Immune System/Microbiota Effects | Modulates gut microbiota; inhibits lymphocyte differentiation and interleukin secretion; suppresses COX-2 and PGE2 expression. | [18] |
Anti-obesity Effects | Promotes brown adipose tissue activation; increases energy expenditure; inhibits lipase, α-glucosidase, and dipeptidyl peptidase-4; reduces triglycerides. | [13] |
Anti-aging Effects | Increases collagen type I expression; inhibits metalloprotease-1; activates SIRT1/mTOR pathway to prevent brain aging. | [36, 37] |
Higher UA levels naturally confer anti-inflammatory, mitochondrial-enhancing, and muscle-strengthening effects. After 8 weeks of UA supplementation at 1 g/day, resistance-trained male athletes showed improvements in muscle strength and endurance. Additionally, UA supplementation was also associated with reduced oxidative stress levels and a decrease in inflammation response levels [16].
A study by García et al. showed that walnut consumption modulated the Gut Microbiota (GM) in a urolithin metabotype-dependent manner within a short period of only three days, and increased the production of Short-Chain Fatty Acids (SCFA) in 27 healthy individuals [43]. Another randomized clinical trial with 66 participants found that urolithin A supplementation was safe and well tolerated. Although, improvements in the 6-minute walking distance and maximal ATP production in the hand muscle were not significant in the urolithin A group vs. the placebo group, long-term urolithin A supplementation was beneficial for muscle endurance and plasma biomarkers, suggesting that urolithin A may counteract age-associated muscle decline [44].
The realization that most individuals do not possess the required microbiome to produce their own Urolithin A (UA) highlights a gap in current nutritional strategies for maintaining a balanced intake of essential compounds from a healthy diet. This underscores the potential of dietary supplementation to compensate for both microbiome deficiencies and variations in dietary habits, that contribute to UA production differences across the population [27].
5. LIMITATIONS, CONFLICTING FINDINGS, AND DOSE-DEPENDENT EFFECTS OF UROLITHINS
Despite promising findings regarding the health benefits of urolithins, several limitations and controversies persist within the research results. These challenges stem from variability in bioavailability, differences in individual gut microbiota composition, inconsistencies in clinical outcomes, and potential dose-dependent effects.
A significant challenge in urolithin research is the considerable inter-individual variability in bioavailability, primarily driven by differences in gut microbiota composition. Only a subset of individuals can efficiently convert ellagitannins into bioactive urolithins, leading to variations in therapeutic efficacy [2, 3]. For instance, individuals classified as metabotype 0 (non-producers) may experience limited or no benefits from urolithin supplementation. This heterogeneity complicates the standardization of dosing regimens, and raises concerns about the generalizability of findings from controlled studies [18].
While numerous in vitro and animal studies have demonstrated the anti-inflammatory, antioxidant, and anticancer properties of urolithins, translating these benefits into clinical settings has proven to be challenging. Some human studies have reported significant improvements in metabolic and cognitive health, whereas others have shown minimal or no effects [4, 17]. Factors contributing to these discrepancies include differences in study design, participant demographics, duration of intervention, and variations in urolithin formulations.
Emerging evidence suggests that urolithins exhibit dose-dependent effects, where lower doses may exert beneficial physiological responses, while higher concentrations could induce cytotoxicity or paradoxical pro-inflammatory effects [22]. For example, while urolithin A has demonstrated neuroprotective and anti-inflammatory benefits at moderate doses, excessive concentrations have been linked to increased oxidative stress and apoptosis in certain cell types [16]. Further research is required to establish optimal dosage ranges for various therapeutic applications to avoid potential adverse effects [36].
The efficacy of urolithins may also be influenced by dietary components that modulate gut microbiota composition or alter metabolic pathways [3]. High-fat diets, for instance, have been shown to impact ellagitannin metabolism, and reduce urolithin bioavailability [1]. Additionally, polyphenol-rich diets containing competing substrates may influence the conversion efficiency of ellagitannins into urolithins, leading to variability in observed health benefits [12].
Most clinical studies evaluating the effects of urolithins have been of short duration, limiting the understanding of their long-term safety and efficacy [33]. There remains a need for well-controlled, long-term human trials to assess potential cumulative effects, interactions with medications, and implications for chronic disease prevention and management [6].
CONCLUSION
While urolithins hold great promise as bioactive compounds with diverse health benefits, several challenges remain. A key limitation is the variability in gut microbiota composition, which influences individual urolithin production and therapeutic responses. The identification of urolithin metabotypes presents a novel framework for precision nutrition and personalized therapy. This review underscores the importance of metabotyping in optimizing urolithin-driven health benefits, particularly for individuals at risk of cardiometabolic, neurodegenerative, and inflammatory diseases.
Clinical evidence suggests that metabotype A individuals experience the most pronounced therapeutic effects, whereas metabotype B and 0 individuals may require microbiome-targeted interventions or direct supplementation. Future research should focus on integrating personalized dietary strategies, microbiome modulation, and clinical biomarkers to enhance urolithin bioavailability and efficacy. Additionally, advancements in novel formulation strategies, such as nanoparticle delivery and liposomal encapsulation, may improve stability and absorption, addressing current bioavailability limitations.
To fully harness the therapeutic potential of urolithins, future studies should establish standardized dosing protocols and investigate long-term safety and efficacy. Expanding research into host-microbiome interactions, metabolic pathways, and dose-response relationships will further refine their application in personalized medicine. This review highlights the need for a multidisciplinary approach, combining clinical nutrition, microbiome science, and pharmacology, to unlock the full potential of urolithins in health and disease management.
AUTHORS’ CONTRIBUTIONS
It is hereby acknowledged that all authors have accepted responsibility for the manuscript's content and consented to its submission. They have meticulously reviewed all results and unanimously approved the final version of the manuscript.
LIST OF ABBREVIATIONS
CVD | = Cardiovascular Disease |
RCT | = Randomized Controlled Trial |
BDNF | = Brain-Derived Neurotrophic Factor |
SCFA | = Short-Chain Fatty Acids |
CONFLICT OF INTEREST
Dr. Vasiliki Lagouri is the Editor In Chief of The Open Medicinal Chemistry Journal.
ACKNOWLEDGEMENTS
Declared none.