All published articles of this journal are available on ScienceDirect.
Kinetic Analysis of Some Chalcones and Synthetic Chalcone Analogues on the Fenton-Reaction Initiated Deoxyribose Degradation Assay1
Abstract
Investigation of in vitro hydroxyl radical scavenging (antioxidant) effect 4-methoxychalcone (1a) and its cyclic analogues (2a-4a), as well as their hydroxyl substituted counterparts (1b-4b) was performed by means of the Fenton-reaction initiated deoxyribose degradation assay in short term (10 minute) and long term (240 minute) experiments. The kinetic deoxyribose method provides possibility to investigate not only the short term antioxidant (hydroxyl radical scavenger) effect but the possible late prooxidant effect of the tested substances as well. In the short term studies compounds 2a, 2b and 4b showed the most pronounced antioxidant effect. The long-term studies showed that the antioxidant activity of all the tested compounds but 4a can be well characterized by the short time determination of the thiobarbituric acid (TBA)-reactive substances (TBARS). Experiments in the presence of ethylenediaminetetraacetic acid (EDTA) resulted in a substantially reduced degradation of deoxyribose in each incubation. Similar to the respective experiment performed without EDTA, the TBARS level of the incubations with 4a showed an increase over the 60-120 minute period. The results demonstrated that complex forming activities that can modify microspeciation and reactivity of iron ions can lead to different short term antioxidant efficiency of the tested substances. Results of the long term incubations indicated that chemical transformation of the tested substances can result formation of derivatives that can initiate further redox activities under the experimental conditions.
INTRODUCTION
Chalcones (1,3-diphenyl-2-propenones) (1) are intermediary compounds of biosynthetic pathway of a very large and widespread group of plant constituents known collectively as flavonoids [1]. Chalcones have been shown to possess antioxidant and anti-inflammatory properties in a variety of experimental systems [2,3]. The compounds have been shown to act as effective cell proliferation inhibitors, anti-tumor promoting and chemopreventive agents [4, 5].
Earlier we have investigated in vitro antineoplastic activity of several synthetic chalcones and the cyclic chalcone analogues, e.g., E-2-(X-benzylidene)-1-indanones (2), -tetralones (3) and benzosuberones (4) (Fig. 1A and 1B). The compounds were evaluated against P388, L1210, Molt 4/C8 and CEM cells as well as against approximately 60 further human tumor cell lines from nine different neoplastic diseases [6-8]. Besides the documented tumor cytotoxic effects, some of the above cyclic chalcone analogues were found to display outstanding CYP1A inhibitor activity [9].
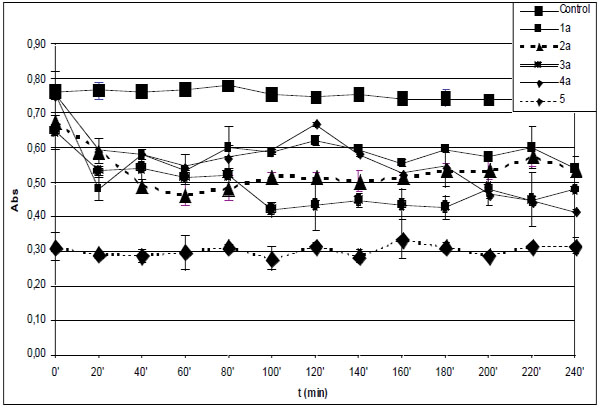
Time-course of degradation of deoxyribose in the presence of 1a-4a and quercetin (5) (200 µM) using 50 µM H2O2.
*Each absorbance (Abs) (λ=532 nm) is the average of three parallel measurements ± standard error. Details of incubation and derivatization is written in the Materials and Methods part.

Time-course of degradation of deoxyribose in the presence of 1b-4b and quercetin (5) (200 µM) using 50 µM H2O2.
*Each absorbance (Abs) (λ=532 nm) is the average of three parallel measurements ± standard error. Details of incubation and derivatization is written in the Materials and Methods part.
Recently, we have investigated the effect of two of the above cyclic chalcone analogues, E-2-(4’-methylbenzylidene)- and E-2-(4’-methoxybenzylidene)-1-benzosuberone (4a) on the cell cycle of Jurkat T cells. It was found that both compounds induced apoptosis and modified the cellular thiol status of the cells [10]. Investigation of effects of 4a, and the hydroxyl-substituted analogs 1b-4b of the methoxy substituted derivatives (1a-4a) on isolated rat liver mitochondria showed the compounds to deplete reduced glutathione (GSH) and disrupt electron transport chain and energetic metabolism. Increased generation of reactive oxygen species (ROS) and possible covalent interaction with GSH was found to be as chemical events accompanied exposure of mitochondria of the investigated compounds [11, 12].
As a continuation of our previous works, in the present paper we report on investigation of in vitro hydroxyl radical scavenging (antioxidant) effect of the previously tested tumor cytotoxic 4-methoxychalcone (1a) and its cyclic analogues 2a-4a, as well as their hydroxyl substituted counterparts (1b-4b) compared to the effect of quercetin (5) (Fig. 1A and 1B). Antioxidant effect of the compounds has been tested by means of the Fenton-reaction initiated deoxyribose degradation assay [13]. Degradation of deoxyribose is supposed to be the result of attack of the Fenton-reaction generated hydroxyl radicals (HO˙), which results in formation of carbonyl fragments [14] that - on heating with TBA at low pH - generate a pink chromogen with absorption maximum at 532 nm [13]. Testing of antioxidant effect (hydroxyl radical-scavenging potential) is based on the competitive reaction of deoxyribose and the tested antioxidant with the reactive hydroxyl radicals [13].
Hydroxyl radicals (HO˙) formed in Fenton-type reactions are reported to react with phenol to generate isomeric dihydroxybenzene derivatives [15]. Since further oxidation (redox cycling) of the formed dihydroxy derivatives could generate reactive oxygen species (ROS), phenolic antioxidants could cause oxidative stress as a result of a series of spontaneous and enzyme catalyzed reactions [16, 17]. Such a mechanism can not be excluded for explanation of the observed prooxidant effect of the previously tested hydroxychalcones (1b-4b) [12]. To test this possibility we applied a modified version of the previously reported kinetic deoxyribose degradation assay [18], which can provide information not only on the immediate antioxidant action but on the possible late prooxidant effect of the investigated compounds as well [19]. Composition of the incubations and details of generation of TBARS were optimized based on the results of Winterbourn [20].
For comparison, we have performed the experiments in the presence of and without addition of ethylenediaminetetraacetic acid (EDTA) to the incubation mixtures. Addition of EDTA results in formation of iron(II)-EDTA complex [21], whose Fenton-activity could result in degradation of EDTA [22]. In the absence of EDTA, a portion of the iron ions is complexed by deoxyribose [23]. In these so called “site-specific” Fenton reactions hydroxyl radicals react immediately at the place where there are formed. Accordingly, compounds with ligand properties compete for the iron ions with the deoxyribose molecules and thus decrease degradation caused by the iron-catalyzed hydroxyl radical attack.
Quercetin (5) has received considerable attention because of its overwhelming presence in foods. The compound may aid in the prevention of several degenerative diseases (e.g., cancer, atherosclerosis, chronic inflammation) by preventing lipid peroxidation [24]. Quercetin (5) is well known as an efficient reducing agent and chelator of transition metals [25]. Scavenging of free radicals and chelation of iron ions both contribute to the antioxidant activity of the compound [23,25].
MATERIALS AND METHODS
Chemicals and Reagents
Synthesis and characterization of the compounds 1a-4a and 1b-4b (Scheme 1) were performed as described earlier [6, 12]. Their structure and purity was checked by their melting points, >1H NMR, TLC (thin layer chromatography) and GC (gas chromatography) methods.
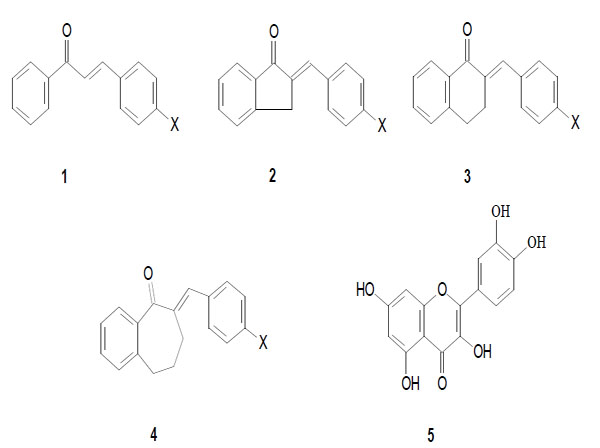
Structure of the investigated chalcones (1), E-2-(X-benzylidene)-1-indanones (2), -tetralones (3) and –benzosuberones (4), and quercetin (5).
Quercetin (5), ethylenediaminetetraacetic acid disodium salt (EDTA), 2-deoxy-D-ribose (deoxyribose), 2-thiobarbituric acid (TBA), dimethyl sulfoxide (DMSO), iron(II) sulfate (FeSO4) and trichloroacetic acid (TCA) were purchased from Sigma-Aldrich (Budapest, Hungary). Other chemicals used were of analytical grade available if otherwise not specified. All solutions were freshly prepared using doubly distilled water. To control the pH value of prepared buffers Mettler Toledo MP 220 pH meter and Mettler Toledo InLab 413 combined electrode was used. The absorbance was recorded with a Specord M40 (Carl Zeiss Jena, Germany) spectrometer. Each value is the average of three independent measurements.
DEOXYRIBOSE DEGRADATION INITIATED BY IRON-DEPENDENT HYDROXYL RADICAL
Deoxyribose Degradation (Control)
The mixture of deoxyribose (9 mM) dissolved in 30 mM phosphate buffer pH 7.4 containing 40 mM sodium chloride, 20 µM/50 µM hydrogen peroxide (H2O2) and 30 µM iron(II) sulphate (FeSO4) was incubated at 37 oC for 10 minutes. The reaction was started by the addition of FeSO4 and degradation of deoxyribose was determined by spectrophotometric measurement of the TBA-reactive substances. The incubation was performed in the presence of 1% (v/v) DMSO, too. The composition of the blanks was the same as above but H2O2 and FeSO4 were omitted.
Deoxyribose Degradation in the Presence of Chalcones (1a,b-4a,b)
The incubations were carried out in the presence (36 µM) or in the absence of EDTA. The reaction mixture contained: 30 mM sodium phosphate buffer pH 7.4 without EDTA or containing 36 µM EDTA, 9 mM deoxyribose, 40 mM sodium chloride, 100 µM or 200 µM chalcone or quercetin (5) dissolved in DMSO corresponding to 1% (v/v) of the total volume of the mixture, 20 µM or 50 µM H2O2, and 30 µM FeSO4. The control mixtures did not contain the investigated compounds, only the vehicle (DMSO). The composition of the blanks was the same as above but H2O2 and FeSO4 were omitted. The reactions were started by addition of FeSO4 and mixtures were incubated at 37°C for 10 minutes and in separate experiments for 4 hours. In these latter cases samples were taken at different (0, 20, 40, 60, 80, 100, 120, 140, 160, 180, 200, and 240 minutes) timepoints. Degradation of deoxyribose was characterized by spectrophotometric determination of the TBA-reactive substances.
Determination of Thiobarbituric Acid Reactive Substances (TBARS)
The thiobarbituric acid reactivity was developed by mixing 1.0 ml aliquot of the incubation mixtures with 0.5 ml thiobarbituric acid reagent (1% (w/v) TBA dissolved in 50 mM sodium hydroxide solution) and 0.5 ml 5.6 % (w/v) TCA followed by heating at 100 oC for 8 minutes. When the mixture was cooled and the developed chromogen was determined by reading the absorbance at 532 nm against appropriate blanks.
RESULTS AND DISCUSSION
Initially, the reaction was investigated using 30 µM iron(II) ion and 20µM H2O2 in the presence of 100 µM chalcone analogues (1a,b-4a,b) using 10 minute incubation time. Under such conditions (excess of iron(II) ions over H2O2) the Fenton reaction is restricted to the quantitative oxidation of iron(II) ions by hydrogen peroxide [26].
Fe2+ + H2O2 = Fe3+ + .OH + OH-
Fe2+ + .OH = Fe3+ + OH-
Accordingly, iron(II) ions, deoxyribose and the tested compounds compete for the forming hydroxyl radicals.
Under such conditions the hydroxyl substituted compound 1b showed the most effective inhibition of deoxyribose degradation (Table 1). Increasing the chalcone concentration to 200 µM resulted in a somewhat more effective antioxidant activity of the investigated compounds. Under these conditions compounds 2a, 2b and 4b showed the most pronounced antioxidant effect (Table 1).
Degradation of Deoxyribose in the Presence of Chalcones (1a,1b), Cyclic Chalcone Analogues (2a,2b,3a,3b,4a,4b) and Quercetin (5) Using 20 µM H2O2; 50 µM H2O2;and 36 µM EDTA + 50 µM H2O2. (Incubation Time: 10 Minute) *
Conditions of the Fenton Reaction | ||||
---|---|---|---|---|
Test Compound | Concentration of test Compound (µM) | 20 µM H2O2 | 50 µM H2O2 | 36 µM EDTA + 50 µM H2O2 |
Absorbance | Absorbance | Absorbance | ||
Control | - | 0,705 ± 0,033 | 0,695 ± 0,003 | 0,356 ± 0,015 |
1a | 100 µM | 0,653 ± 0,028 | 0,613 ± 0,007 | 0,274 ± 0,014 |
1a | 200 µM | 0,592 ± 0,041 | 0,615 ± 0,017 | 0,255 ± 0,014 |
2a | 100 µM | 0,701 ± 0,032 | 0,674 ± 0,028 | 0,310 ± 0,035 |
2a | 200 µM | 0,554 ± 0,029 | 0,557 ± 0,006 | 0,293 ± 0,016 |
3a | 100 µM | 0,652 ± 0,032 | 0,681 ± 0,023 | 0,298 ± 0,001 |
3a | 200 µM | 0,573 ± 0,017 | 0,617 ± 0,012 | 0,231 ± 0,006 |
4a | 100 µM | 0,699 ± 0,018 | 0,635 ± 0,055 | 0,336 ± 0,038 |
4a | 200 µM | 0,631 ± 0,017 | 0,561 ± 0,001 | 0,245 ± 0,029 |
1b | 100 µM | 0,571 ± 0,032 | 0,644 ± 0,061 | 0,243 ± 0,037 |
1b | 200 µM | 0,550 ± 0,013 | 0,528 ± 0,011 | 0,236 ± 0,027 |
2b | 100 µM | 0,622 ± 0,019 | 0,621 ± 0,030 | 0,274± 0,004 |
2b | 200 µM | 0,531 ± 0,011 | 0,538 ± 0,014 | 0,248 ± 0,002 |
3b | 100 µM | 0,671 ± 0,019 | 0,677 ± 0,001 | 0,280 ± 0,028 |
3b | 200 µM | 0,553 ± 0,012 | 0,651 ± 0,012 | 0,259 ± 0,018 |
4b | 100 µM | 0,690 ± 0,028 | 0,653 ± 0,031 | 0,326 ± 0,017 |
4b | 200 µM | 0,516 ± 0,019 | 0,541 ± 0,005 | 0,231 ± 0,033 |
5 | 100 µM | 0,349 ± 0,044 | 0,351 ± 0,001 | 0,218 ± 0,015 |
5 | 200 µM | 0,246 ± 0,031 | 0,255 ± 0,011 | 0,160 ± 0,007 |
* Each absorbance (λ=532 nm) is the average of three parallel measurements ± standard error. Details of incubation and derivatization is written in the Materials and Methods part.
Increasing the H2O2 concentration to 50 µM (excess of H2O2 over iron(II) ions) did not drastically change either the degree of oxidation of deoxyribose in the control samples or the antioxidant effect of the investigated compounds. Comparison of the corresponding results of the 20 µM and 50 µM H2O2 experiments showed a small but statistically significant increase of antioxidant effect of 1a and 4a used in 100 µM concentration. Results of the two series of investigations showed the hydroxyl substituted 1b-4b to have somewhat more effective antioxidant activities. Quercetin (5) was statistically more effective antioxidant than any of the investigated chalcones under both investigated conditions (Table 1).
Performing the latter experiments in the presence of 36 µM EDTA resulted in a substantially reduced degradation of deoxyribose even in the control samples (Table 1). Reduced degradation of deoxyribose can be rationalized by the presence of the strong iron chelator EDTA [21, 22]. At the same time the relative antioxidant activity of almost all the investigated compounds but the reference 5 increased in both concentrations. This observation suggest that complexation of iron ions by quercetin (5) - which can be substantially reduced by EDTA - plays an important role of antioxidant effect of 5 under the EDTA-free experimental conditions. In the presence of the strong iron chelator EDTA such effect of quercetin is diminished and its antioxidant (hydroxyl radical scavenger) activity becomes similar to that of the structurally related chalcones. The highest increase of antioxidant effect at the elevated (200 µM) concentration was observed in the case of the benzosuberone derivatives 4a and 4b, and the tetralone derivative 3a. Quercetin (5), the most effective tested compound, was also found to be more effective in the 200 µM concentration (Table 1).
Time course of the Fenton-reaction initiated deoxyribose degradation was investigated using 50 µM H2O2 and 200 µM test compounds without or in the presence of EDTA over a 240 minute time period. Application of long term incubation under such conditions (excess of H2O2 over iron(II) ions) provides the possibility to investigate whether chemical modification of the tested compounds by the Fenton reaction generated hydroxyl radicals results in formation of redox active derivatives which can initiate redox cycling (involving reduction of the iron(III) to iron(II) ions) and generation of hydroxyl radicals over the time of the extended incubation period [16]. On the other hand, reduction of iron(III) to iron (II) ions can diminish the iron(III)-catalyzed –non hydroxyl radical mediated- degradation of deoxyribose, which might be responsible for formation of some of the TBARS during the boiling phase of the method [27].
In the experiments performed without EDTA the level of TBA-reactive substances (TBARS) in the control incubations was found to be almost constant over the 240 minute period (Fig. 1A and B). This observation suggests that the initially formed TBARS and iron(III)-levels are stable under the control’s conditions. According to the expectations, the initial antioxidant activity of the tested compounds could be well characterized by the short time determination of TBARS. Comparison of levels of TBARS of the control incubations at the 10 minute (ABS=0.705) (Table 1) and the 20 minute (ABS=0.765) (Fig. 1A) timepoints suggests that most of the Fenton-reaction initiated degradation of deoxyribose is taking place in the first 10 minutes of incubation. The zero minute level of TBARS (ABS=0.761) is very much the same as that of the 20 minute level (Fig. 1A) due to the heating of the freshly mixed samples at 100 oC to obtain the TBA chromogen. It is also worth mentioning that the zero minute level of TBARS is rather close to the control level in the case of all investigated compounds but 5 (Fig. 2B and B). This latter observation can be considered as the result of formation of iron-quercetin (5) complex [28] which results in reduced extent of deoxyribose degradation in “site-specific” degradation reactions.
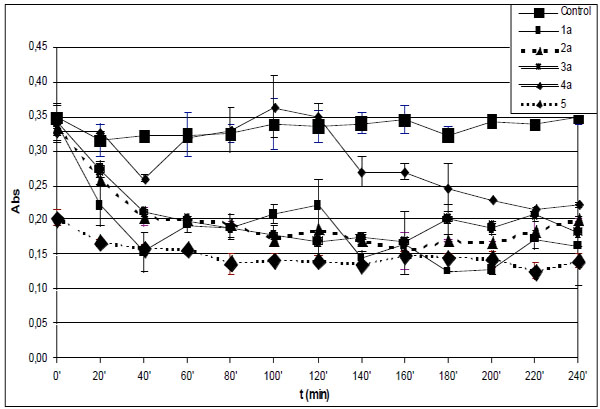
Time-course of degradation of deoxyribose in the presence of 1a-4a and quercetin (5) (200 µM) using 36 µM EDTA and 50 µM H2O2.
*Each absorbance (Abs) (λ=532 nm) is the average of three parallel measurements ± standard error. Details of incubation and derivatization is written in the Materials and Methods part.
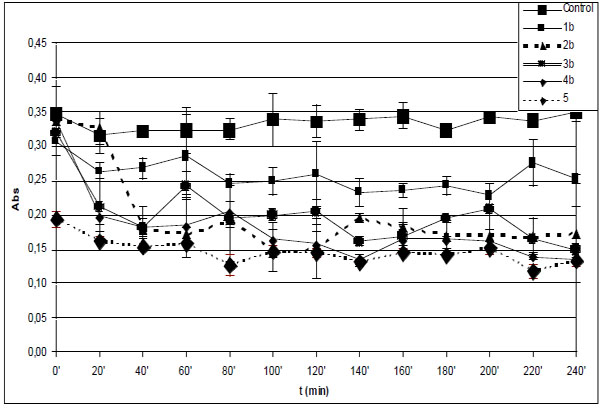
Time-course of degradation of deoxyribose in the presence of 1b-4b and quercetin (5) (200 µM) using 36 µM EDTA and 50 µM H2O2.
*Each absorbance (Abs) (λ=532 nm) is the average of three parallel measurements ± standard error. Details of incubation and derivatization is written in the Materials and Methods part.
Among the hydroxyl substituted compounds 1b-4b the indanone derivative 2b was found to be the most effective antioxidant (Fig. 1B). The TBARS level remained almost constant for each investigated compound from the 20 minute timepoint over the 240 minute period (Fig. 1B). On the contrary, the TBARS levels in the incubation mixtures of the methoxy substituted analogues 1a-4a slightly changed during the prolonged incubation time (Fig. 1A). For example, 3a and 4a displayed a significantly reduced TBARS levels after the 80 minute and 120 minute timepoints, respectively. These observations suggest redox activities that result in decrease of the TBA-reactive derivatives in the incubation systems.
Fenton-reaction initiated degradation of deoxyribose of the control incubations in the presence of EDTA (36 µM) resulted in significantly lower level of TBARS than that of without EDTA. Similar to the experiments performed without EDTA, the level of TBARS in the control incubations was found to be almost constant over the 240 minute period (Fig. 2A and B). Under such conditions the zero time level of TBARS is also very much the same in all incubations but in that performed in the presence of 5, where it is statistically lower.
The TBARS levels in the incubations of the hydroxyl substituted 1b-4b performed in the presence of EDTA are comparable to that of the reference 5 in each case but the open-chain 1b (Fig. 2B). This observation also underlines importance of formation of iron(II)-quercetin (5) complex in the significantly higher antioxidant effect of 5 under the EDTA-free experimental conditions. Similar investigation of the methoxy substituted 1a-4a showed all the incubations but that with 4a to have TBARS levels similar to that of with 5 (Fig. 2A). It was found that the TBARS level of the incubation with 4a showed an increase over the 60-120 minute period followed by significant decrease over the rest of the incubation time (Fig. 2A). In order to get a better understanding of the observed prooxidant effect of 4a ethyl acetate extract of the 120 minute incubation sample was investigated by GC-MS. GC-MS analysis of the ethyl acetate extract could not indicate presence of any derivative of the parent compound. Further studies are needed to provide experiment-based explanation of this observation. Such a prooxidant activity of 4a is characteristically different from the results obtained with the respective hydroxyl substituted 4b.
In summary it was demonstrated that scope of the original “test-tube” assay for evaluation of antioxidant properties of potential antioxidants based on the degree of Fenton-reaction initiated degradation of deoxyribose is valid but limited. Although the initial antioxidant activity of the tested compounds can be well characterized by the short time determination of TBA-reactive substances, complex forming activities that can modify microspeciation and reactivity of iron ions can lead to different antioxidant efficiency of the tested substances. Chemical modification of the tested substances can result formation of derivatives that can initiate further redox activities under specific experimental conditions that can convert short-term “antioxidants” to “prooxidants” on longer observation time. To have a more comprehensive evaluation of antioxidant/prooxidant activities of xenobiotics long term investigations are recommended with/without of transition metal chelating agents (e.g., EDTA) that can modify microspeciation of iron ions. On the other hand, the added chelating agents can also act as competitive hydroxyl radical scavengers in the Fenton-type test systems.
NOTES
1 The paper is considered as Part V of the series E-2-Benzylidenecyclanones. Part IV: P. Perjési, J. Linnanto, E. Kolehmainen, E. Osz, E. Virtanen, J. Mol. Struct. 2005, 740 81-89..
ACKNOWLEDGEMENT
This study was supported by the Faculty of Medicine Research Fund (AOK-KA 34039-12/2009), University of Pécs. The authors express their sincere thanks to Szilárd Molnár (South-Transdanubian Environmental Protection Inspectorate, H-7634 Pécs-Cserkút, Hungary) for performing the GC-MS measurements in his laboratory, and to Zsuzsanna Moravecz (University of Pécs) for her excellent technical assistance.