Dose-Dependent Tissue Distribution of K117, a Bis-pyridinium Aldoxime, in Rats
Abstract
Background:
Bis-pyridinium aldoximes are reactivators of the paraoxon-inhibited butyrylcholinesterase enzyme. Paraoxon is the active product of parathion, a widely used insecticide.
Objective:
The objective of this study is to examine the dose-dependent distribution of K117, a bis-pyridinium aldoxime in rat tissues.
Materials and Methods:
White male Wistar rats were intramuscularly injected with various doses of K117; the animals were sacrificed 30 minutes after injections. The dose-dependent body distribution of K117 was determined using reversed-phase HPLC.
Results:
Dose-dependent distribution of K117 in body tissues was linear in the serum and other body tissues throughout the whole range of the concentrations studied. However, the of distribution was not observed in the brain and cerebrospinal fluid, especially with high doses.
Conclusion:
The body distribution of K117 significantly depends on doses used, the p-value is: 500 nmol, i.m., when applied in the range of 100 to 10,000 nmol.
1. INTRODUCTION
There are two types of cholinesterase enzymes in humans and animals. The acetylcholinesterase enzyme (AcChE, EC 3.1.1.7) is located in many tissues (red blood cell membranes, the central nervous system, peripheral organs, cholinergic and non-cholinergic fibers etc.) and functions postsynaptically with an extremely high catalytic activity at nerve synapses to terminate the effect of acetylcholine by its hydrolyzation.
The butyrylcholinesterase enzyme (BuChE, EC 3.1.1.8), also known as pseudocholinesterase or plasma esterase, serves as a backup for AcChE [1]. It is responsible for the quick inactivation through the hydrolysis of different endogenous and exogenous esters in the blood plasma. The potential poisons of AcChE are scavenged by BuChE, and the human body has BuChE (approximately 680 nanomoles) about tenfold as much as AcChE [2]. BuChE also plays en essential role in metabolizing cocaine, heroin, mivacurium, succinylcholine and procaine. BuChE-deficiency results in increased sensitivity to succinylcholine (a widely used depolarizing neuromuscular blocking agent in clinical practice). It is generally accepted that BuChE has an essential role in the inactivation of toxic compounds including organophosphorous esters. Measuring BuChE serves as a biomarker for organophosphate exposure [3]. Either AcChE- or BuChE-deficiency is an indicator of a special depression that is common in pesticide handlers [4].
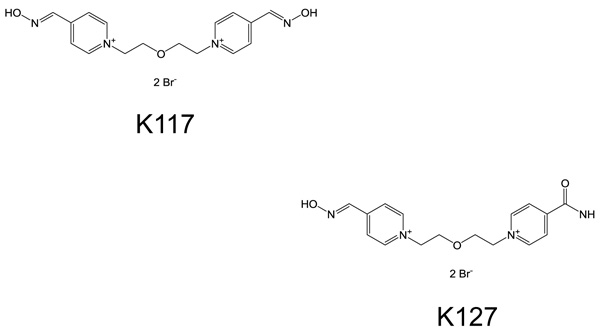
Reactivation of AcChE- or BuChE-deficiency caused by insecticide poisoning is crucial to the survival of a poisoned patient. Karasova et al. [5] reported that both K117 and K127 were among the best reactivators of BuChE, when some currently available and several newly synthesized pyridinium aldoximes were studied on rats intoxicated by tabun. The reactivation efficiency of K117, and also that of HI-6, obidoxime, triedoxime, K127, K206, K250, K251, K269, K347, K628, were experimentally determined in plasma and the brain by Kovarik et al. [6]. K117 showed similar effectivity as HI-6 in peripheral tissues. Jun et al. [7] compared the reactivation potency of several pyridinium aldoximes in vitro on paraoxon-inhibited human AcChE and BuChE. Two of the reactivators (obidoxime and trimedoxime) worked well on inhibited AcChE, giving a reactivation rate over 75% when used in a concentration of 100 µM concentration. However, none of the classical pyridinium aldoximes (pralidoxime, methoxime, obidoxime, trimedoxime, HI-6) produced the reactivation rate of BuChE over 10%, even when they were used in a concentration of 100 µM. Kuca et al. [5, 7, 8] found that K117 and atropine co-doses work efficiently in vitro on rats intoxicated by tabun.
Sakurada et al. [9, 10] were the first to detect and measure that pralidoxime penetrated through the blood-brain barrier. Similar determinations and statements were made by Kalász et al. [11, 12], who expanded the analysis of pyridinium aldoximes on the cerebrospinal fluid.
This paper presents a dose-dependent tissue penetration of the K117 from the site of its intramuscular application to various target organs.
2. MATERIALS AND METHODS
2.1. Chemicals and Solvents
All solvents and chemicals were bought from commercial sources in the best possible quality. Pyridinium aldoximes (K117 and K127) were supplied by the Department of Chemistry, University of Hradec Kralove, Czech Republic. The chemical structures of these two compounds are given in Fig. (1).
2.2. Animals and Animal Treatment
White male Wistar rats weighing 180-199 grams were obtained from Toxicoop (Budapest, Hungary). Two animals were treated intramuscularly (i.m.) with an adequate dose of a freshly prepared aqueous solution of K117 (0.1, 0.3, 1.0, 3.0 and 10.0 µmol for each pair of rats). The rats were sacrificed 30 minutes after treatment, keeping the ethical regulation of Semmelweis University. Body fluids (serum, cerebrospinal fluid) were taken and certain organs/tissues (brain, eyes, lungs, testes, liver, kidneys and inner ear) were dissected and treated with perchloric acid, homogenized and centrifuged. HPLC determinations of K117 were done using K127 as an internal standard (Figs. 2 and 3), as detailed in our previous publication [13].
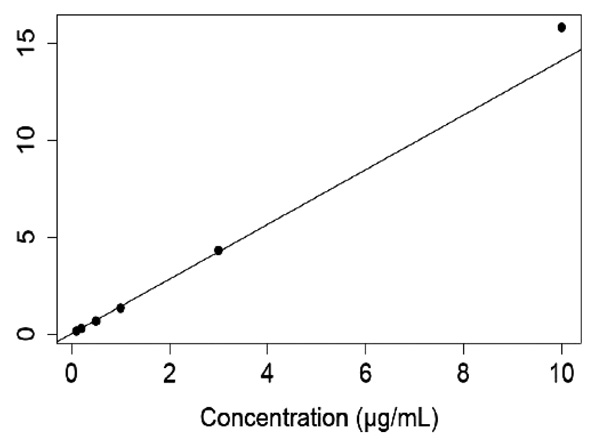
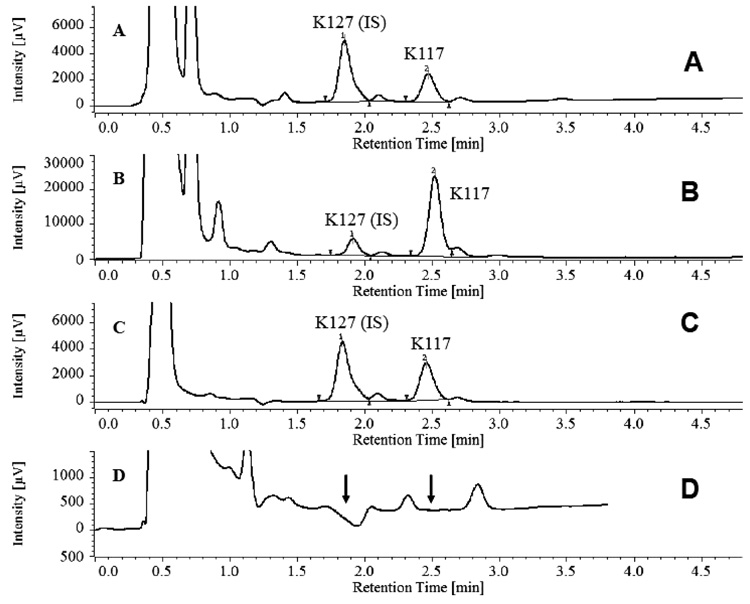
Sample (tissue or body fluid) | Concentration (µg/ml± SD) Determined by HPLC-UV | Tissue Concentration (ng/ml) | Tissue concentration related to the serum concentration (%) | Concentration. related to the dose of 1 µmol | |
---|---|---|---|---|---|
Dose: 0.1 µmol | |||||
Serum | 0.011±0.005 | 110 | 100.0 | 0.567 | |
Brain | 0.011±0.005 | 55 | 50.00 | 3.235 | |
CSF | 0.010±0.000 | 50 | 45.45 | 5.880 | |
Eyes | 0.010±0.002 | 50 | 45.45 | 0.990 | |
Lung | 0.055±0.004 | 275 | 250.0 | 2.350 | |
Testis | 0.006±0.004 | 30 | 27.27 | 5.450 | |
Liver | 0.043±0.005 | 215 | 195.5 | 39.09 | |
Kidney | 0.340±0.012 | 1700 | 1545 | 42.50 | |
Inner ear | 0.079±0.005 | 395 | 359.0 | 3.361 | |
Dose: 0.3 µmol | |||||
Serum | 0.022±0.008 | 220 | 100.0 | 0.378 | |
Brain | 0.015±0.004 | 75 | 34.09 | 1.470 | |
CSF | 0.012±0.003 | 60 | 27.27 | 2.352 | |
Eyes | 0.014±0.005 | 70 | 31.82 | 0.460 | |
Lung | 0.051±0.002 | 255 | 115.9 | 0.720 | |
Testis | 0.009±0.004 | 45 | 20.45 | 2.727 | |
Liver | 0.077±0.027 | 385 | 175.0 | 3.208 | |
Kidney, | 0.768±0.009 | 3840 | 1745 | 0.920 | |
Inner ear | 0.088±0.010 | 440 | 200.0 | 0.838 | |
Dose: 1.0 µmol | |||||
Serum | 0.194±0.003 | 1940 | 100.0 | 1.00 | |
Brain | 0.034±0.003 | 170 | 8.762 | 1.00 | |
CSF | 0.017±0.011 | 85 | 4.381 | 1.00 | |
Eyes | 0.101±0.013 | 505 | 26.03 | 1.00 | |
Lung | 0.234±0.006 | 1170 | 60.30 | 1.00 | |
Testis | 0.011±0.004 | 55 | 2.835 | 1.00 | |
Liver | 0.080±0.010 | 400 | 20.61 | 1.00 | |
Kidney, | 2.781±0.026 | 13905 | 716.7 | 1.00 | |
Inner ear | 0.235±0.006 | 1175 | 60.56 | 1.00 | |
Dose: 3.0 µmol | |||||
Serum | 0.576±0.013 | 5760 | 100.0 | 0.989 | |
Brain | 0.083±0.006 | 415 | 7.204 | 0.813 | |
CSF | 0.023±0.016 | 115 | 1.996 | 0.451 | |
Eyes | 0.222±0.005 | 1111 | 19.28 | 0.733 | |
Lung | 0.601±0.013 | 3005 | 52.17 | 0.856 | |
Testis | 0.093±0.009 | 465 | 8.072 | 2.818 | |
Liver | 0.194±0.020 | 970 | 16.84 | 0.808 | |
Kidney | 4.698±0.159 | 23490 | 407.8 | 0.563 | |
Inner ear | 0.442±0.064 | 2210 | 38.36 | 0.626 | |
Dose: 10.0 µmol | |||||
Serum | 2.289±0.121 | 22890 | 100.0 | 1.179 | |
Brain | 0.360±0.004 | 1800 | 7.863 | 1.058 | |
CSF | 0.075±0.004 | 375 | 1.638 | 0.441 | |
Eyes | 0.763±0.035 | 3815 | 16.66 | 0.755 | |
Lung | 1.788±0.033 | 8940 | 39.05 | 1.770 | |
Testis | 0.484±0.003 | 2420 | 10.57 | 4.400 | |
Liver | 0.153±0.002 | 765 | 3.342 | 0.190 | |
Kidney | 11.219±0.099 | 56095 | 245.06 | 0.403 | |
Inner ear | 1.246±0.047 | 6230 | 27.21 | 0.530 |
3. RESULTS
Dose-dependence of K117 levels in various body compartments of rats is given in Table 1.
Table 1 shows very high levels of K117 in the lungs, liver, kidney and Inner ear compared to those in serum. These relatively high levels continuously decrease with time in the lungs and inner ear, while they continue to increase in the liver and kidney.
4. DISCUSSION
During their in vitro experiments, Jun et al. [7] compared AcChE and BuChE reactivating process of several K-compounds against paraoxon-inhibition, using a concentration as high as 100 µM, as the overall (in vivo) toxicity does not limit the dose used. However, studying the dose-dependence in in vivo experiments injections up to 100 µM could be applied, as higher doses of K117 were toxic [6]. Horn et al. [14] experimentally proved that K117 fulfils one of the basic requirements of an adequate antidote, it does not even influence the enzyme activity of BuChE in excess (1,000 µM). Thereby, K117 belongs to the group of pyridinium aldoximes that can be potentially used (K27, K48, K74, K75, K99, K127, K203, etc.) in medical practice. The BuChE reactivation power of K117 is preferable in paraoxon-inhibited BuChE, while its (in vitro) activity on tabun-inhibited enzyme is not significant.
It is the circulating blood that supplies K117 from the site of i.m. injection to each organ, tisssue and cell of rats. However, special barriers of the organism (e.g. blood-brain barrier, blood-testis barrier etc.) can either totally or partially hinder the transfer of K117 to special organs such as the central nervous system and the organs of reproduction. Each biological barrier has its own characteristics. When brain concentrations are compared to the serum levels of K117 no proportionality can be observed. About 50% of K117 could penetrate into the brain when a dose of 0.1 µmol was given. However, this ratio decreased to 9% when 1 µmol and to 7% when 10 µmol K117 were applied, respectively. This dynamic function of the blood-brain-barrier was even more expressed for CSF; at a dose of 0.1 µmol. The relative concentration of K117 in the CSF compared to that in the serum, was 45%, however, this ratio decreased to 5% and 1.6% using doses of 1 µmol and 10 µmol, respectively. Lorke et al. [15] also demonstrated dynamic changes in blood-brain barrier and blood-CSF barrier functions. The relatively high proportion of K-117 in the kidneys, 30 minutes following intramuscular administration, compared to serum and the liver versus serum, indicates the essential role of the kidneys in the removal of K117 from the organism. It also indicates that K117 is hydrophilic and, therefore, is excreted from the body via the kidney.
The inner ear has a multi-compartmental structure (peri-, endolymph) with several different barrier systems (e.g. blood-endolymph, blood-perilymph, CSF-perilymph) [16, 17], which makes the explanation of the relatively high K117 concentrations in the inner ear difficult. Experimental data suggest that a much slower elimination from the perilymph [18, 19] is presumably a major factor in the development of substance accumulation in the inner ear.
CONCLUSION
The analysis of tissue concentrations of K117 levels relative to serum concentrations is a useful method to determine the special characteristics of the penetration of this compound into critically important organs and tissues. The validated reversed-phase HPLC bioanalytical method developed was sensitive and selective enough for detailed pharmacokinetic measurements.
ETHICS APPROVAL AND CONSENT TO PARTICIPATE
All animal experiments were carried out according to the ethical requirements of Semmelweis University (permission number of Governmental Office of Pest County, Hungary, PE/EA/385-5/2018.)
HUMAN AND ANIMAL RIGHTS
No human were used in the study. Al the procedures followed were in accordance with the standards set forth in the eighth edition of “Guide for the Care and Use of Laboratory Animals” (grants.nih.gov/grants/olaw/guide-for-the-care-and-use-of-laboratory-animals_prepub.pdf published by the National Academy of Sciences, The National Academies Press, Washington, D.C.).
CONSENT FOR PUBLICATION
Not applicable.
CONFLICT OF INTEREST
The authors declare no conflict of interest, financial or otherwise.
ACKNOWLEDGEMENTS
This project is based on a Corsorcium Agreement between Korea Research Institute (Daejeon, Korea), Jagiellonian University (Krakow, Poland), University of Hradec Kralove (Hradec Kralove, Czech Republic) and Semmelweis University (Budapest, Hungary).
The authors express their thanks to Mr. János Horváth and Ms. Bogi Szalacsi for their advice. Technical assistance was provided by Ms. Györgyike Guth, Ms. Krisztina Kecskés, Mr. Zoltán Szimrók and Mr. András Keglevich.
This project was financially supported by the grant NN126968 of National Research, Development and Innovation Office (NRDIO, Budapest, Hungary) this research was supported by Ministry of Education, Youth and Sports of the Czech Republic (No. 8F17004) via V4-Korea Joint Research Program and also by Kalász Teaching and Research Co. (Budapest, Hungary). Grant No. K128875 of NRDIO served as partial source of salary to JSz.