Inhibition of PPARγ by Natural Compounds as a Promising Strategy in Obesity and Diabetes
Abstract
A wide group of natural compounds (flavonoids, stilbenes, neolignans and others) has been identified as Peroxisome Proliferator-Activated Receptor (PPAR) agonists, with a large variety of chemical structure and different activity versus the three PPAR subtypes. These receptors are transcription factors controlling metabolic pathways in the organism, involved in lipid and glucose metabolism, cell differentiation and energy homeostasis. Otherwise, very little is known about natural compounds able to inhibit PPARs. A number of studies demonstrate that PPARγ repression has a beneficial effect in reducing body weight and improving insulin sensitivity, suggesting a potential clinical role in obesity and type 2 diabetes. This review analyzes natural compounds able to repress PPAR activity and their potential use in metabolic disorders.
1. INTRODUCTION
Obesity is considered one of the major metabolic disease in industrialized countries, being the number of obese always increasing worldwide. Sedentary life, incorrect lifestyles and high-fat diet contribute to the weight gain, in addition to hereditary and medical factors. This disease is strongly linked to concomitant medical situations, as dyslipidemia, hypertension, coronary heart disease, type 2 diabetes and metabolic syndrome. For these reasons, there is an urgent need for drugs able to contrast obesity and related metabolic disorders.
Peroxisome Proliferator-Activated Receptors (PPARs), members of the nuclear receptor superfamily, represent important transcription factors controlling different metabolic pathways in the organism [1]. They are involved in lipid and glucose metabolism, in cell differentiation and energy homeostasis [2]. The three PPAR isoforms, PPARα, PPARγ, and PPARδ, encoded by distinct genes, present a different distribution, related to specific metabolic actions in liver, adipose tissue, skeletal muscle, kidney and heart [3]. PPARα activation promotes the fatty acid catabolism, by lowering triglyceride levels and rising HDL cholesterol; it represents the molecular target of fibrates, hypolipidemic drugs, used in dyslipidemia and hypertriglyceridemias [4]. PPARγ is a key regulator of glucose homeostasis, promoting adipocyte differentiation, insulin sensitivity, and glucose utilization in tissues [5]. PPARδ, the most ubiquitous PPAR isoform, plays important metabolic roles by controlling the expression of genes involved in fatty acid uptake, β-oxidation and energy utilization [6].
Since some decades, the Thiazolidinediones (TZDs), PPARγ agonists, have been used as antidiabetic drugs, being able to improve insulin sensitivity [7]. Despite their beneficial effects in the treatment of diabetic patients, major safety problems emerged during the treatment with TZDs, including hepatic dysfunction, hypoglycemia, weight gain, cardiovascular failure and edema; for these reasons, some compounds of this class were withdrawn from the pharmaceutical market or discontinued from clinical studies [8, 9]. Many research efforts have been made by researchers to discover novel and potent PPARγ agonists, in an attempt to maximize the efficacy and minimize side effects [10, 11].
An alternative strategy, developed in recent years, aims at obtaining a reduced activation of PPARs: in fact, some experimental studies showed reduced body weight and improved insulin sensitivity, suggesting a potential clinical role of PPARγ antagonists in obesity and type 2 diabetes [12-14]. Likewise, promising biological effects were demonstrated by reduced activation of PPARα and PPARδ, mainly in different cancer models [15-21].
The rising interest in the moderate activation of PPARs was directed by researchers also in the natural compound field: the nature historically offers a wide range of precious active ingredients, utilized by traditional medicine. Literature surveys describe a very large number of natural compounds able to activate PPARs, mainly PPARγ subtype: stilbenes, flavonoids, neolignans, sesquiterpenes, amorfrutins, coumarins and several others (Fig. 1) [22, 23]. In addition, the incorporation of different natural scaffolds, such as stilbenes and chalcones, afforded novel synthetic molecules endowed with a PPAR agonist activity [24-27].
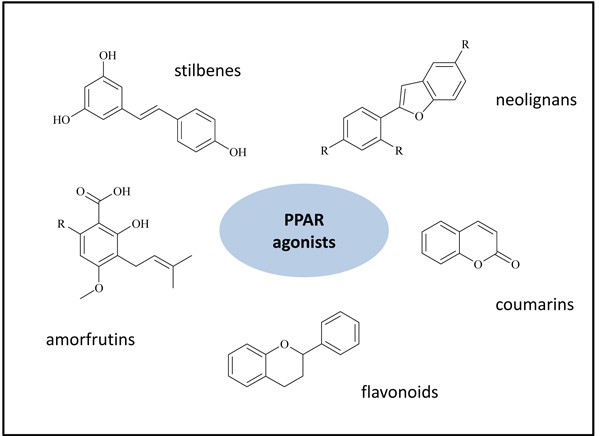
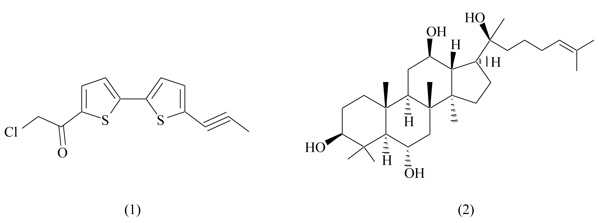
Compared to agonists, only few natural compounds have been identified with antagonistic activity on PPARs: in particular, to the best of our knowledge, natural PPARα and PPARδ antagonists are not mentioned in literature, while a short group of PPARγ antagonists has been recently reported.
PPARγ antagonists from natural sources will be the focus of this review, with special attention to their promising activities as antiobesity and antidiabetic agents.
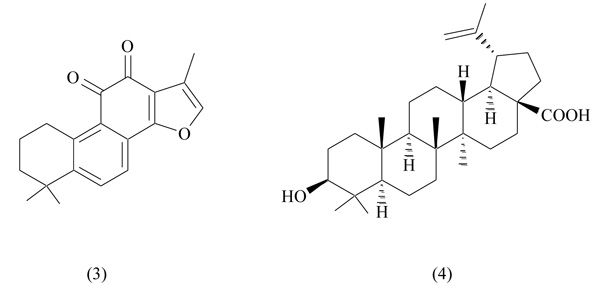
2. PPARγ ANTAGONISTS FROM NATURAL SOURCES
Several natural compounds have been identified as PPAR activators, with a large variety of chemical structure and different activity versus the three PPAR subtypes. Many of them are PPARγ agonists, generally showing activity in the low micromolar range. From the structural point of view, there is a great variability, with flavonoids, stilbenes, neolignans, polyacetylenes, amorfrutins and others. Otherwise, very little is known about natural compounds able to inhibit PPARs. Compounds mentioned in the literature show a repressive effect on PPARγ, whereas no data are available on selective PPARα and PPARδ natural antagonists.
In the next paragraphs, natural PPARγ antagonists will be analyzed, focusing on their promising antiobesity and antidiabetic properties.
2.1. 7-Chloroarctinone-b
7-Chloroarctinone-b, isolated from the roots of Rhaponticum uniflorum (Asteraceae family), is an ethynyl-thiophene derivative able to inhibit PPARγ activation (Fig. 2) [28]. This plant is widely distributed in China and has been long used in traditional medicine for the treatment of fever and intoxications. Several pharmacological effects were described for ethynyl-thiophenes, including antitumor, antifungal and antiviral activities.
7-Chloroarctinone-b is a selective PPARγ antagonist, capable of inhibiting rosiglitazone-induced transcriptional activity in a dose-dependent way (IC50 5.61 µM) [29]. After binding to PPARγ, 7-chloroarctinone-b antagonizes the heterodimerization PPAR/RXR, necessary to start the transcription machinery. Additionally, the same study demonstrates the ability of 7-chloroarctinone-b to inhibit the recruitment of the PPARγ coactivator CBP (cAMP response element binding protein). This compound significantly blocked the differentiation of adipocytes 3T3-L1 in a dose-dependent manner [29].
2.2. Protopanaxatriol
Ginseng has been largely used as food and tonic herb for the treatment of various pathological conditions, including obesity and diabetes, mainly in Asiatic countries [30]. The reduction of body weight induced by ginseng and its constituents in obese animal models makes this plant a promising candidate for metabolic diseases; however, molecular mechanisms involved in these therapeutic effects remain unclear to date [31, 32].
Protopanaxatriol (PPT, Fig. 2), extracted from roots of Panax ginseng, is a major constituent of this plant, and it shows anti-carcinogenic effects in in vitro and in vivo models [33]. A recent study identified the protopanaxatriol as a PPARγ antagonist with moderate binding affinity [34]. In a competitive binding assay using TR-FRET, PPT induced a selective antagonism on PPARγ (IC50 11.75 µM), showing no activity versus other nuclear receptors controlling metabolic functions, as PPARα, PPARδ and LXR. PPT induced a significant inhibition of adipocyte differentiation (3T3-L1) and a repressive effect on genes involved in adipogenesis, as PPARγ, adipose fatty acid-binding protein 2 (aP2), Cluster of Differentiation 36 (CD36), Lipoprotein Lipase (LPL) and Fatty Acid Synthase (FAS) [34]. In vivo experiments on C57BL/6 obese mice confirmed previous results: PPT treatment ameliorates metabolic disorders, reducing body weight and fasting blood glucose levels, and improving insulin resistance [34]. These scientific evidences suggest the potential use of protopanaxatriol as a dietary supplement for the treatment of obesity.
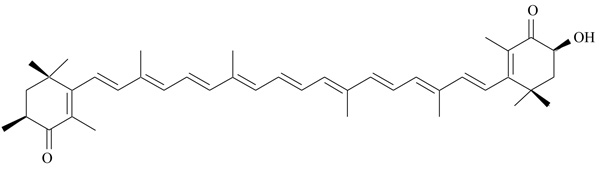
2.3. Tanshinone IIA
Tanshinone IIA represents one of the most abundant constituents of the roots of Salvia miltiorrhiza (danshen), a plant distributed in Asiatic countries, widely used in Chinese traditional medicine to treat cardiovascular and cerebrovascular diseases [35]. Tanshinone IIA, a lipophilic diterpene (Fig. 3), is available as sulfonate salt for use in angina, stroke, cardiovascular disorders and ischemic attacks. The therapeutic effects of danshen have been attributed to the antioxidant and anti-inflammatory activity of tanshinone IIA. In addition, recently, it has been reported a beneficial effect of this natural compound in weight suppression and adipocyte differentiation [36].
Tanshinone IIA has been identified as a PPARγ antagonist through a competitive binding assay: it was able to displace radiolabeled rosiglitazone, showing a moderate binding affinity (IC50 3.897 µM) [37]. In in vitro experiments tanshinone IIA strongly inhibited adipocyte differentiation (3T3-L1) in a dose-dependent manner; in obese mice, it decreased adipose mass and body weight, improved glucose tolerance and positively affected serum lipid levels. These beneficial properties make this natural compound potentially useful in obesity and related metabolic disorders.
2.4. Betulinic Acid
Betulinic acid (3β-Hydroxy-lup-20(29)-en-28-oic acid) (Fig. 3), a pentacyclic triterpene found in the bark of several plants, shows a wide range of biological activities, including antiviral, anti-inflammatory, antimalarial and antitumor [38, 39]. In a murine model of obesity, this compound inhibited adipogenesis, preventing abdominal fat accumulation: this result suggests an antiobesity potential for betulinic acid [40]. In a recent work by Brusotti et al betulinic acid, extracted from the African plant Diospyros bipindensis, was identified as PPARγ and PPARα antagonist [41]. It was able to displace rosiglitazone from PPARγ (IC50 3.1 µM) and Wy-14643 from PPARα (IC507.4 µM) in a luciferase-based transactivation assay. The crystal structure of the complex PPARγ-betulinic acid was obtained, showing a unique binding mode, accounting for antagonistic properties of the ligand. In vitro studies on adipocytes (3T3-L1) and osteoblasts (MC3T3-E1) showed a marked decrease of adipogenesis, a promotion of osteogenesis and an improvement of glucose uptake [41]. These preliminary results are of great interest and confirm the pharmacological potential of betulinic acid in different pathologies.
2.5. Astaxanthin
Astaxanthin is a natural carotenoid, found in a great variety of red-colored aquatic organisms, as salmon, crustaceans and microalgae (Fig. 4) [42]. It is structurally similar to beta-carotene, but it does not work as a precursor of vitamin A in the human organism. Thanks to its antioxidant activity, it is mainly used as a dietary supplement for human consumption, but also as a food colorant. Astaxanthin protects against lipid peroxidation and contrasts the oxidative damage of cells and tissues; its antiatherogenic effects were studied in animal models of cardiovascular diseases [43]. Additional beneficial activity of astaxanthin has been described in different studies, where it demonstrated hypolipidemic and antiatherogenic effects [44, 45]. In high cholesterol diet fed rats, astaxanthin induced a marked decrease of total cholesterol, Low-density Lipoprotein Cholesterol (LDL-C), Very Low-density Lipoprotein Cholesterol (VLDL-C) and triglycerides, and increased High-density Lipoprotein Cholesterol (HDL-C). A significant reduction in atherosclerotic lesions was observed on aorta of high cholesterol-fed rats, after treatment with astaxanthin [45]. The effects of astaxanthin on serum lipids prompted researchers to investigate about a possible mechanism of action involving PPAR receptors; the study by Jia and coworkers showed that astaxanthin works as moderate PPARα agonist (EC50 3.9 µM) and PPARγ antagonist (IC50 607.8 µM), whereas it is inactive versus PPARδ [46]. These findings were confirmed analyzing the expression profile of specific target genes for PPARα and PPARγ. In lipid-loaded hepatocytes, the treatment with astaxanthin produced a strong reduction of cellular lipid accumulation: these data support the potential of astaxanthin as nutritional prevention of obesity and metabolic disorders.
2.6. Foenumoside B
Lysimachia foenum-graecum is an herbal plant, used as a spice and insect repellent. In traditional oriental medicine, the extract of this plant has been used for the treatment of various disorders, as cold, headache, toothache, discomforts of the digestive system. Different triterpene saponins, named foenumoside A-E, have been identified as active ingredients, and a wide panel of biological effects have been described, including antioxidant, anti-inflammatory, and antiadipogenic [47, 48]. The antiadipogenic effect was attributed to Foenumoside B (Fig. 5), but the mechanism of action at the molecular level was not determined [49]. A recent study examined a possible connection between Foenumoside B and Lysimachia foenum-graecum extract with PPARγ, a master regulator of adipocyte differentiation [50]. In transactivation assays, both Foenumoside B and Lysimachia foenum-graecum extract showed a selective antagonism on PPARγ, with IC50 7.63 and 22.5 µg/mL, respectively. They strongly affected adipocyte differentiation induced by PPARγ agonists, and reduced expression of lipogenic genes. In vivo administration of Lysimachia foenum-graecum extract in ob/ob and KKAy mice induced beneficial effects on body weight, insulin resistance and glucose tolerance, offering novel therapeutic strategies in the treatment of obesity, insulin resistance and metabolic disorders.
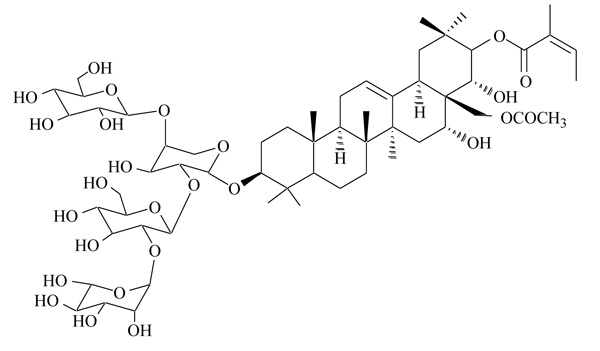
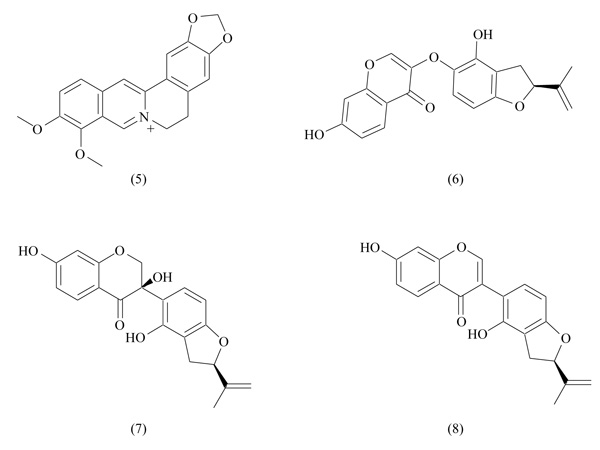
3. PPARγ INHIBITORS FROM NATURAL SOURCES
A number of natural compounds affect PPAR activity, inducing beneficial effects in adipocyte differentiation, insulin sensitivity and glucose and lipid homeostasis. However, sometimes the inhibition of PPAR is not related to an antagonistic effect, but it may be induced by multiple mechanisms affecting PPAR levels and activity. Some compounds were reported as PPAR inhibitors, but it was not effectively demonstrated their binding to the receptor. The observed repression of PPAR could be ascribed to a gene downregulation of PPAR expression, not related to a classical antagonism on the receptor. Berberine and isoprenyl dihydrofuran isoflavonoids are example of compounds inhibiting PPARγ, but not acting as antagonists.
Berberine is an active alkaloid extracted from Berberis vulgaris, found also in other plants as Cortidis rhizome. Given the multiple pharmacological actions of berberine, it was used in Chinese traditional medicine to treat diarrhea, dysentery, stomatitis, hepatitis [51]. From a structural point of view, berberine belongs to the class of protoberberines, showing an isoquinoline core (Fig. 6). Multiple beneficial effects of berberine attracted the attention of scientists, and several studies were carried out to clarify its pharmacological and therapeutic effects. Berberine has shown antihyperlipidemic and antihyperglycemic effects, increased insulin secretion and sensitivity, suggesting a potential application in the prevention or treat-ment of diabetes [52, 53]; anti-inflammatory and antitumor effects were also described [54, 55]. Given the multiple meta-bolic effects played by berberine, its PPAR activity was investigated: results confirmed that metabolic actions of berberine are mediated by PPARs [56]. Berberine showed inhibitor activity on PPARγ and PPARα, but it did not induce transcriptional activity changes: these data indicate that berberine does not act as an antagonist, and the PPAR inhibition is probably a consequence of the reduction of PPAR protein levels. Moreover, berberine inhibited adipocyte differentiation and proliferation, acting also on target genes involved in adipocyte differentiation [56].
In analogy to berberine, some isoprenyl dihydrofuran isoflavonoids (Fig. 6) extracted from Crotalaria albida have been identified as adipocyte differentiation inhibitors through downregulation of PPARγ activity [57]. In transactivation assays, these compounds strongly inhibited rosiglitazone-induced PPARγ activity. They induced suppression of adipocyte differentiation and adipogenesis, probably mediated by PPARγ. However, there is no real evidence about the binding of these compounds with PPARγ; for this reason, they have been indicated as PPARγ inhibitors, and not as antagonists.
CONCLUSION AND PERSPECTIVES
Since centuries ago and still to date, plants have been offered to humans precious ingredients with various pharmacological properties. The use of plants in traditional medicine accounts for the wide panel of beneficial effects they can induce in humans. Natural compounds identified in the past were used in medicine or served as lead compounds, useful for the discovery of novel synthetic drugs.
Among the great variety of pharmacological effects, many plants show antiobesity and antidiabetic effects, suppressing adipocyte differentiation and adipogenesis. In light of these evidences, many researchers studied the mechanisms involved in these activities: in particular, the role of PPARγ was explored, being this receptor a key regulator of metabolic functions in the cells. For a number of plants, it was demonstrated that these effects were effectively linked to a reduction of PPARγ activity.
This review has been focused on natural compounds showing an antagonistic behavior on PPARγ, and their potential as antiobesity and antidiabetic drugs. Moreover, if a great number of PPAR agonists have been obtained from natural sources, very little is known about natural PPAR antagonists. Compounds analyzed in this review showed the ability to inhibit adipocyte differentiation and proliferation, reduce the weight gain, improve the insulin sensitivity and glucose tolerance. Indeed, results obtained in in vitro and in vivo experiments are really encouraging, but they require further studies to clarify a possible utilization in humans for the treatment of obesity, diabetes and related metabolic disorders. Some of these natural compounds are currently used as dietary supplement, given their general safety and the lacking of important side effects.
Whereas some natural compounds were demonstrated PPARγ antagonists, for some compounds a PPAR repression was reported, but it was not effectively demonstrated the binding to the receptor. The observed repression of PPAR could be ascribed to a gene downregulation of PPAR protein expression, also if the compound does not produce an antagonism on the receptor. Compounds acting in this way, as berberine and some isoflavonoids, were reported as PPAR inhibitors, and not as PPAR antagonists.
To the best of our knowledge, natural compounds able to selectively inhibit PPARα and PPARδ have not been identified to date.
LIST OF ABBREVIATIONS
PPARs | = Peroxisome Proliferator-Activated Receptors |
TZDs | = Thiazolidinediones |
RXR | = Retinoid X Receptor |
CBP | = cAMP response element binding protein |
PPT | = Protopanaxatriol |
TR-FRET | = Time-resolved Fluorescence Resonance Energy Transfer |
LXR | = Liver X Receptor |
aP2 | = Adipose fatty acid-binding Protein 2 |
CD36 | = Cluster of Differentiation 36 |
LPL | = Lipoprotein Lipase |
FAS | = Fatty Acid Synthase |
LDL-C | = Low Density Lipoprotein Cholesterol |
VLDL-C | = Very Low Density Lipoprotein Cholesterol |
HDL-C | = High Density Lipoprotein Cholesterol |
CONSENT FOR PUBLICATION
Not applicable.
CONFLICT OF INTEREST
The authors declare no conflict of interest, financial or otherwise.
ACKNOWLEDGEMENTS
Declared none.