All published articles of this journal are available on ScienceDirect.
Anti-BVDV Activity Evaluation of Naphthoimidazole Derivatives Compared with Parental Imidazoquinoline Compounds
Abstract
Background:
Pestivirus genus includes animal pathogens which are involved in economic impact for the livestock industry. Among others, Bovine Viral Diarrhoea Virus (BVDV) establish a persistent infection in cattle causing a long list of symptoms and a high mortality rate. In the last decades, we synthesised and reported a certain number of anti-BVDV compounds.
Methods:
In them, imidazoquinoline derivatives turned out as the most active. Their mechanism of actions has been deeply investigated, BVDV RNA-dependent RNA polymerase (RpRd) resulted as target and the way of binding was predicted in silico through three main H-bond interaction with the target.
The prediction could be confirmed by target or ligand mutation. The first approach has already been performed and published confirming the in silico prediction.
Results:
Here, we present how the ligand chemical modification affects the anti-BVDV activity. The designed compounds were synthesised and tested against BVDV as in silico assay negative control.
Conclusion:
The antiviral results confirmed the predicted mechanism of action, as the newly synthesised compounds resulted not active in the in vitro BVDV infection inhibition.
1. INTRODUCTION
Bovine Viral Diarrhoea Virus (BVDV) is the prototype of the Pestivirus genus of the Flaviviridae family. The Pestivirus genus also contains other animal pathogens such as the Bovine Viral Diarrhoea Virus (BVDV) [1], the Border Disease Virus (BDV) and the Classical Swine Fever Virus (CSFV). BVDV causes infertility [2, 3] in cattle but also teratogenesis, early embryonic death, abortion, respiratory problems and immune system disorder on cows which results in acute infections of immunocompetent cattle causing a mortality rate of 17 to 32% [4] and an estimated loss between 10 and 40 million $ per million calvings [5, 6]. The virus was also considered to be a valuable surrogate for the Hepatitis C Virus (HCV) in antiviral drug studies [7]. During the years, several mammalians from different species have been infected by BVDV [8], which was not host-specific. BVDV can also be a problematic contaminant in the laboratory, BVDV strains have been identified in commercially available lots of foetal bovine serum and cell lines [9], and therefore in interferons and vaccines for medical use [10, 11]. Several conventional live and killed vaccines in the years have been developed, but they showed non-efficacy or safety issues [12-14]. No antivirals are currently available for controlling BVDV infections in laboratories or farms, only voluntary BVDV control programmes [15] seemed to be successful, but BVDV remains an agronomical burden, hence the antiviral research on molecules that can specifically inhibit the virus replication could be useful to control outbreaks in farms. In the last decades, a certain number of selective anti-BVDV compounds have been reported, this includes virus targeting and host targeting derivatives, e.g. polymerase inhibitors [7, 16-18], protease inhibitors [19] and human cellular enzymes inhibitors [20-22]. The antiviral research against animal pathogens of the Pestivirus genus is still a challenge. In the framework of a long-lasting antiviral research program, our group designed and synthesised a series of angular and linear N-polycyclic derivatives active against BVDV, YFV, HCV and other related viruses [23-30]. The molecular target for BVDV inhibition was identified in the viral RNA dependent RNA polymerase (RdRp) [24, 28, 31, 32]. Imidazo[4,5-g]quinoline derivatives were published as interesting BVDV inhibitors and the most active derivative of that series, 4-(4-chloro-3H-imidazo[4,5-g]quinolin-2-yl)benzonitrile (A) (EC50 0.3µM; CC50 >100 µM) [32, 33] was docked and scored for affinity towards the binding site of the BVDV RdRp, the resulting receptor/ligand complex was relaxed by energy minimisation, followed by MD simulation. The naphthoimidazole core showed significant H-bond interaction between nitrogen atoms and key amino acids (R295, Y674 and S411). In detail, arginine 295 interacts with N5 of the lead compound as H-bond donor, while N1 resulted as H-bond acceptor in the interaction with tyrosine 674 and the nitrogen of the benzonitrile moiety gives H-bond with serine 411. Has also been predicted the decrease of affinity of some molecules with the substrate when R295 and Y674 – critical residues for compound binding - are mutated into alanine and the prediction has been confirmed by in vitro assays on the mutant RdRp [32]. In order to have a further negative control for these simulations, we now designed some chemical modification on the lead compounds and parent compounds similarly active in BVDV RdRp inhibition, and we synthesised the designed compounds. The replacement of the nitrogen atom in position 5 of imidazo [4,5-g] quinoline derivatives with a carbon atom, resulted in naphtho [2,3-d] imidazole derivatives (3a-j) designed to confirm the essential interaction between N5 and the arginine 295. The 2-phenyl moiety was modified in order to assess the relevance of interactions with serine 411.
2. MATERIALS AND METHODS
2.1. Synthetic Methods
Synthesis of derivatives 3a-j was carried on by using the general procedure for imidazole ring closure as described in literature [34] by mixing commercial naphthalene-2,3-diamine (1) and corresponding commercial aldehydes (2a-j) in a ratio 1:1 dissolved in acetonitrile (CH3CN), added with H2O2 30% (ratio 1:7) and HCl 37% (ratio 1:3,5) at room temperature overnight, solid was obtained (Scheme. I). The solid has been filtered off with vacuum, washed with acetonitrile first and with water then, till neutral pH of the filtrate. The crude solid has been stored and dried in the oven overnight and were obtained pure by crystallisation from ethanol.
2.2. Chemical Characterisation
Compounds melting points (m.p.) were taken in open capillaries in a Köfler hot stage or Digital Electrothermal melting point apparatus and are uncorrected. Retention factors (Rf) were measured by Thin Layer Chromatography (TLC) using Merck F-254 commercial plates and a proper mixture of petroleum spirit (PS) and ethyl acetate (EA) as eluent. Nuclear Magnetic Resonance (NMR) spectra were registered in solutions in deuterated DMSO and were recorded with a Bruker Avance III 400 NanoBay (400 MHz) instrument. 1H-NMR chemical shifts are reported in parts per million (ppm) downfield from tetramethylsilane (TMS) used as internal standard. Chemical shift values are reported in ppm (δ) and coupling constants (J) are reported in Hertz (Hz). Signal multiplicities are represented as s (singlet), d (doublet), dd (doublet of doublets), ddd (doublet of doublet of doublets), t (triplet), td (triplet of doublets), q (quadruplet) and m (multiplet). The assignment of exchangeable protons (OH and NH) was confirmed by the addition of D2O. 13C-NMR chemical shifts are reported in downfield from tetramethylsilane (TMS) used as internal standard. Suitable method among APT (Attached Proton Test) and jmod (J-modulated spin-echo for X-nuclei coupled to H-1 to determine the number of attached protons) was selected for each compound. The solutions for ESI-MS measurements were prepared by dissolving the solid compounds in HPLC acetonitrile to obtain a concentration of 1.0-2.0 ppm. Mass spectra in the positive-ion mode were obtained on a Q Exactive Plus Hybrid Quadrupole-Orbitrap (Thermo Fisher Scientific) mass spectrometer. The solutions were infused at a flow rate of 5.00 μl/min into the ESI chamber. The spectra were recorded in the m/z range 150–800 at a resolution of 140 000 and accumulated for at least 2 min in order to increase the signal-to-noise ratio. The instrumental conditions used for the measurements were as follows: spray voltage 2300 V, capillary temperature 250 °C, sheath gas 10 (arbitrary units), auxiliary gas 3 (arbitrary units), sweep gas 0 (arbitrary units), and probe heater temperature 50 °C. ESI-MS spectra were analysed by using Thermo Xcalibur 3.0.63 software (Thermo Fisher Scientific), and the average deconvoluted monoisotopic masses were obtained through the Xtract tool integrated with the software.
2.3. Cells and Viruses
Cell lines were purchased from American Type Culture Collection (ATCC). The absence of mycoplasma contamination was checked periodically by the Hoechst staining method. Cell line supporting the multiplication of BVDV was the following: Madin-Darby Bovine Kidney (MDBK) [ATCC CCL 22 (NBL-1) Bos Taurus]. The virus was purchased from American Type Culture Collection (ATCC), Bovine Viral Diarrhoea Virus (BVDV) [strain NADL (ATCC VR-534)].
2.4. Cytotoxicity Assays
Cytotoxicity assays were run in parallel with antiviral assays. MDBK cells were seeded at an initial density of 6x105 cells/cm3 in 96-well plates, in culture medium [Minimum Essential Medium with Earle’s salts (MEM-E) with L-glutamine, supplemented with 10% horse serum and 1 mM sodium pyruvate, and 0.025 mg/L kanamycin]. Cell cultures were then incubated at 37 °C in a humidified, 5% CO2 atmosphere in the absence or presence of serial dilutions of test compounds. Cell viability was determined after 48–96 h at 37 °C by the 3-(4,5-dimethylthiazol-2-yl)-2,5- diphenyl- tetrazolium bromide (MTT) method [35].
2.5. Antiviral Assay
Compounds activity against BVDV was based on inhibition of virus-induced cytopathogenicity in MDBK cells acutely infected with an m.o.i. of 0.01. Briefly, MDBK cells were seeded in 96-well plates at a density of 3x104 cells/well and were allowed to form confluent monolayers by incubating overnight in growth medium at 37 °C in a humidified CO2 (5%) atmosphere. Cell monolayers were then infected with 50 µL of virus dilution in maintenance medium (MEM-E with L-glutamine, supplemented with 0.5% inactivated FBS, 1 mM sodium pyruvate and 0.025 g/L kanamycin) to give an m.o.i of 0.01. After 2 h, 50 µL of maintenance medium, without or with serial dilutions of test compounds, were added. After a 3 days incubation at 37 °C, cell viability was determined by the MTT method. Linear regression analysis: viral and cell growth at each drug concentration was expressed as a percentage of untreated controls and concentrations, resulting in 50% (EC50 and CC50) growth inhibition. NM 108 (2’-C-methylguanosine) and ribavirin are used as reference controls.
2.6. Experimental Section
2.6.1. 2-phenyl-1H-naphtho[2,3-d]imidazole (3a)
Compound 3a (C17H12N2, MW 244.291) was obtained in total yield 95%; m.p. 254.6-255 °C; TLC (PS/EA 6/4): Rf 0.55. 1H-NMR (DMSO-d6): δ 8.49 (2H, d, J= 6.8 Hz, H-2’,6’), 8.37 (2H, s, H-4,9), 8.19 (2H, dd, J= 3.2 Hz, H-5,8), 7.77 (3H, m, H-3’,4’,5’), 7.56 (2H, dd, J= 3.2 Hz, H-6,7). 13C-NMR (APT, DMSO-d6): δ 152.9 (C-2), 133.7 (CH-4), 132.4 (C-9a), 130.9 (3C, C-3a,4a,8a), 129.6 (3CH, C-3’,4’,5’), 128.6 (2CH, C-2’,6’), 128.1 (2CH, C-6,7), 125.4 (2CH, C-5,8), 123.8 (C-1’), 110.7 (CH-9). ESI-MS (m/z): calcd. for C17H12N2 245.1073, found 245.1072 [M+H]+.
2.6.2. 2-(p-tolyl)-1H-naphtho[2,3-d]imidazole (3b)
Compound 3b (C18H14N2, MW 258.317) was obtained in total yield 74%; m.p. 276.6-278 °C; TLC (PS/EA 6/4): Rf 0.62. 1H-NMR (DMSO-d6): δ 8.35 (2H, s, H-4,9), 8.26 (2H, d, J= 8 Hz, H-2’,6’), 8.19 (2H, dd, J= 3.2 Hz, H-5,8), 7.57 (4H, m, H-6,7,3’,5’), 2.47 (3H, s, CH3). 13C-NMR (APT, DMSO-d6): δ 152.9 (C-2), 144.6 (C-3a), 131.9 (C-4’), 130.9 (2C, C-4a,8a), 130.2 (2CH, C-3’,5’), 128.5 (2CH, C-2’,6’), 128.0 (2CH, C-6,7), 126.3 (C-9a), 125.5 (2CH, C-5,8), 120.6 (C-1’), 110.5 (2CH, C-4,9), 21.3 (CH3). ESI-MS (m/z): calcd. for C18H14N3 259.1230, found 259.1231 [M+H]+.
2.6.3. 2-(4-nitrophenyl)-1H-naphtho[2,3-d]imidazole (3c)
Compound 3c (C17H11N3O2) was obtained in total yield 72%; m.p. 236-238 °C; TLC (PS/EA 6/4): Rf 0.63. 1H-NMR (DMSO-d6): δ 8.57 (2H, d, J= 8.8 Hz, H-3’,5’), 8.51 (2H, d, J= 8.8 Hz, H-2’,6’), 8.28 (2H, s, H-4,9), 8.10 (2H, q, J= 3.6 Hz, H-5,8), 7.47 (2H, q, J= 3.2 Hz, H-6,7). 13C-NMR (APT, DMSO-d6): δ 152.5 (C-2), 148.8 (C-3a), 137.8 (C-4’), 133.9 (C-9a), 130.6 (2C, C-4a,8a), 128.4 (2CH, C-6,7), 127.9 (2CH, C-5,8), 127.3 (C-1’), 124.4 (2CH, C-2’,6’), 124.3 (2CH, C-3’,5’), 111.5 (2CH, C-4,9). ESI-MS (m/z): calcd. for C17H11N3O2 290.0924, found 290.0922 [M+H]+.
2.6.4. 4-(1H-naphtho[2,3-d]imidazol-2-yl)benzonitrile (3d)
Compound 3d (C18H11N3, MW 269.300) was obtained in total yield 85%; m.p. 237.7-239.1 °C; TLC (PS/EA 6/4): Rf 0.58. 1H-NMR (DMSO-d6): δ 8.53 (2H, d, J= 8.4 Hz, H-3’,5’), 8.29 (2H, s, H-4,9), 8.17 (2H, d, J= 8 Hz, H-2’,6’), 8.12 (2H, dd, J= 3.2 Hz, H-5,8), 7.49 (2H, dd, J= 3.2 Hz, H-6,7). 13C-NMR (APT, DMSO-d6): δ 152.5 (C-2), 136.6 (C-3a), 133.2 (2CH, C-3’,5’), 131.3 (C-9a), 130.7 (2C, C-4a,8a), 128.3 (2CH, C-2’,6’), 127.9 (2CH, C-6,7), 124.5 (2CH, C-5,8), 118.2 (CN), 113.8 (C-4’), 111.3 (2CH, C-4,9). ESI-MS (m/z): calcd. for C18H11N3 270.1026, found 270.1025 [M+H]+.
2.6.5. 2-(4-(trifluoromethyl)phenyl)-1H-naphtho[2,3-d]imida- zole (3e)
Compound 3e (C18H11F3N2, MW 312.289) was obtained in total yield 89%; m.p. 211.3-212 °C; TLC (PS/EA 6/4): Rf 0.78. 1H-NMR (DMSO-d6): δ 8.66 (2H, d, J= 8 Hz, H-2’,6’), 8.38 (2H, s, H-4,9), 8.17 (2H, dd, J= 3.2 Hz, H-5,9), 8.14 (2H, d, J= 8.4 Hz, H-3’,5’), 7.54 (2H, dd, J= 3.2 Hz, H-6,7). 13C-NMR (APT, DMSO-d6): δ 151.6 (C-2), 133.0 (C-3a), 132.4 (1C, m, CF3), 131.0 (2C-4a,8a), 129.3 (2CH, C-2’,6’), 128.0 (2CH, C-6,7), 127.1 (C-9a), 126.4 (CH-5), 125.4 (2CH, C-3’,5’), 125.0 (C-4’), 123.4 (CH-8), 122.3 (C-1’), 111.0 (2CH, C-4,9). ESI-MS (m/z): calcd. for C18H11F3N2 313.0947, found 313.0946 [M+H]+.
2.6.6. 2-(4-methoxyphenyl)-1H-naphtho[2,3-d]imidazole (3f)
Compound 3f (C18H14N2O, MW 274.317) was obtained in total yield 91%; m.p. 251-252.2 °C; TLC (PS/EA 6/4): Rf 0.40. 1H-NMR (DMSO-d6): δ 8.40 (2H, d, J= 8.8 Hz, H-2’,6’), 8.32 (2H, s, H-4,9), 8.19 (2H, dd, J= 3.2 Hz, H-5,8), 7.57 (2H, dd, J= 3.2 Hz, H-6,7), 7.35 (2H, d, J= 8.8 Hz, H-3’,5’), 3.94 (3H, s, CH3). 13C-NMR (APT, DMSO-d6): δ 163.7 (C-4’), 152.9 (C-2), 132.1 (2C, C-3a,9a), 130.9 (3C, C-4a,8a,1’), 130.6 (2CH, C-2’,6’), 128.0 (2CH, C-6,7), 125.4 (2CH, C-5,8), 115.3 (2CH, C-3’,5’), 110.2 (2CH, C-4,9), 55.9 (CH3). ESI-MS (m/z): calcd. for C18H14N2O 275.1179, found 275.1176 [M+H]+.
2.6.7. 2-(5-nitrofuran-2-yl)-1H-naphtho[2,3-d]imidazole (3g)
Compound 3g (C15H9N3O3, MW 279.250) was obtained in total yield 79%; m.p. 217-217.4 °C; TLC (PS/EA 6/4): Rf 0.46. 1H-NMR (DMSO-d6): δ 8.19 (2H, s, H-4,9), 8.05 (2H, dd, J= 3.2 Hz, H-5,8), 7.93 (1H, d, J= 3.6 Hz, H-4’), 7.68 (1H, d, J= 3.6, H-3’), 7.42 (2H, dd, J= 3.2 Hz, H-6,7). 13C-NMR (jmod, DMSO-d6): δ 152.1 (C-5’), 146.6 (C-2’), 144.7 (C-2), 138.9 (2C, C-3a,9a), 130.6 (2C, C-4a,8a), 127.8 (2CH, C-6,7), 124.0 (2CH, C-5,8), 114.8 (2CH, C-3’,4’), 111.80 (2CH, C-4,9). ESI-MS (m/z): calcd. for C15H9N3O3 280.0717, found 280.0717 [M+H]+.
2.6.8. 2-(5-nitrothiophen-2-yl)-1H-naphtho[2,3-d]imidazole (3h)
Compound 3h (C15H9N3O2S, MW 295.316) was obtained in total yield 71%; m.p. 253-254.1 °C; TLC (PS/EA 6/4): Rf 0.54. 1H-NMR (DMSO-d6): δ 8.28 (1H, d, J= 4.4 Hz, H-4’), 8.18 (2H, s, H-4,9), 8.12 (1H, d, J= 4.4 Hz, H-3’), 8.04 (2H, dd, J= 3.2 Hz, H-5,8), 7.42 (2H, dd, J= 3.2 Hz, H-6,7). 13C-NMR (APT, DMSO-d6+TFA-d): δ 153.1 (C-5’), 149.2 (C-2), 147.4 (C-3a), 139.0 (C-9a), 136.4 (C-2’), 130.8 (2C, C-4a,8a), 130.4 (CH-3’), 129.2 (CH-4’), 1270.8 (2CH, C-6,7), 124.6 (2CH, C-5,8), 111.3 (2CH, C-4,9). ESI-MS (m/z): calcd. for C15H9N3O2S 296.0488, found 296.0489 [M+H]+.
2.6.9. 2-(pyridin-4-yl)-1H-naphtho[2,3-d]imidazole (3i)
Compound 3i (C16H11N3, MW 245.279) was obtained in total yield 85%; m.p. 250.9-251.6 °C; TLC (PS/EA 6/4): Rf 0.11. 1H-NMR (DMSO-d6): δ 9.03 (2H, d, J= 6 Hz, H-3’,5’), 8.60 (2H, d, J= 5.6 Hz, H-2’,6’), 8.31 (2H, s, H-4,9), 8.09 (2H, dd, J= 3.2 Hz, H-5,8), 7.46 (2H, dd, J= 3.2 Hz, H-6,7). 13C-NMR (APT, DMSO-d6): δ 151.0 (C-2), 146.6 (2CH, C-2’6’), 139.7 (C-3a), 138.0 (C-9a), 130.8 (2C, C-4a,8a), 128.0 (2CH, C-6,7), 127.5 (C-4’), 124.5 (2CH, C-5,8), 122.6 (2CH, C-3’,5’), 112.0 (2CH, C-4,9). ESI-MS (m/z): calcd. for C16H11N3 246.1026, found 246.1025 [M+H]+.
2.6.10. 2-(pyridin-2-yl)-1H-naphtho[2,3-d]imidazole (3j)
Compound 3j (C16H11N3, MW 245.279) was obtained in total yield 65%; m.p. 238.3-239 °C; TLC (PS/EA 6/4): Rf 0.20. 1H-NMR (DMSO-d6): δ 8.79 (1H, d, J= 4.8 Hz, H-3’), 8.46 (1H, d, J= 8 Hz, H-6’), 8.16 (2H, s, H-4,8), 8.05 (1H, td, 1J= 7.6 Hz, 2J= 1.6 Hz, H-5’), 8.01 (2H, dd, J= 3.2 Hz, H-5,8), 7.59 (1H, ddd, 1J= 7.6 Hz, 2J= 4.8 Hz, 3J= 1.2 Hz, H-4’), 7.38 (2H, dd, J= 3.2 Hz). 13C-NMR (APT, DMSO-d6): δ 155.2 (C-2), 150.1 (CH-6’), 148.4 (C-2’), 138.2 (CH-4’), 137.1 (C-3a), 130.7 (2C, C-4a,8a), 129.1 (C-9a), 128.3 (2CH, C-6,7), 126.0 (CH-3’), 124.1 (2CH, C-5,8), 122.2 (2CH, C-4,9), 108.7 (CH-5’). ESI-MS (m/z): calcd. for C16H11N3 246.1026, found 246.1026 [M+H]+.
3. RESULTS AND DISCUSSION
As described above, the driven idea for this project is the isosteric substitution of a nitrogen atom with a carbon atom and evaluate the antiviral activity of the resulted molecules compared with the parental compounds.
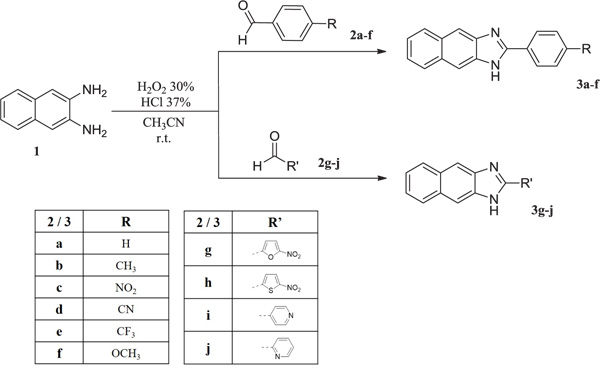
Compound | MDBK (aCC50) | BVDV (bEC50) |
---|---|---|
3a | 37.5±3.5 | 16.2±2.6 |
3b | 11.6 | >11.6 |
3c | >100 | >100 |
3d | >100 | >100 |
3e | 11.8 | >11.8 |
3f | 2.8 | >2.8 |
3g | >100 | >100 |
3h | 4.7 | >4.7 |
3i | >100 | >100 |
3j | >100 | >100 |
A | >100 | 0.3 |
NM 108 | >100 | 1.5±0.2 |
Ribavirin | >100 | 18±2 |
bCompound concentration (µM) required to achieve 50% protection of MDBK cells from the BVDV-induced cytopathogenicity, as determined by the MTT method.
Naphthoimidazole derivatives ( 3a - j ) were designed and synthesised in order to confirm the relevance of nitrogen atom in position 5 of the imidazoquinoline derivatives for the interaction with the target, these molecule’s antiviral results confirmed the predicted affinity data previously published since they showed a consistent loss of antiviral activity with respect to parental compounds [32]. Derivative 3a still maintains a weak antiviral activity, EC50 at 16 µM, but related at cytotoxicity CC50 value of 37 µM. Cytotoxicity is consistent in the greater part of analysed compounds, contrary to what is detected for parental imidazoquinoline derivatives, 3b, 3e, 3f, 3h presented CC50 values ranging between 2.8 and 11.8 µM, (Table 1). Derivative 3d, which is as much like compound A, revealed a total loss of activity and, therefore, loss of affinity with the target. Clearly, the interactions between the aromatic moiety - here labelled as phenyl-R or R’ - and serine 411 of the target RdRp are not responsible for the anti-BVDV activity and are only supportive to it.
CONCLUSION
The naphthoimidazole derivatives were designed and synthesized as isostere of imidazoquinoline derivatives (previously reported) to evaluate the interactions between heteroatoms of the chemical scaffold of these derivatives and the key amino acids of the BVDV RdRp. The quinoline nitrogen atom was substituted with a carbon atom, which cannot make H-bond interactions with R295 amino acid, and this resulted in total loss of activity, as showed by the antiviral assay results. We can then confirm the predicted relevance of N5 - R295 interaction from the docking studies published. This isosteric substitution led to not active derivatives against BVDV. At the same time, the biochemical interaction between the aromatic moiety - here labelled as R - and serine 411 of the RdRp target is clearly not responsible but only bearing, for the anti-BVDV activity.
ETHICAL APPROVAL AND CONSENT TO PARTICIPATE
Not applicable.
HUMAN RIGHTS AND ANIMAL RIGHTS
No humans or Animals were the basis of this research.
CONSENT FOR PUBLICATION
Not applicable.
FUNDING
This study is supported by (P.O.R. Sardegna F.S.E. - Operational Programme of the Autonomous Region of Sardinia, European Social Fund 2014-2020 - Axis III Education and training, Thematic goal 10, Investment Priority 10ii), Specific goal 10.5.
CONFLICT OF INTEREST
The authors declare no conflict of interest, financial or otherwise.
ACKNOWLEDGEMENTS
Declared none.