All published articles of this journal are available on ScienceDirect.
A Review on The Medicinal And Industrial Applications of N-Containing Heterocycles
Abstract
Nitrogen-containing heterocycles constitute an important division of organic chemistry. The structural and functional diversity in nitrogen-containing heterocyclic compounds emanates from the presence and nature of the heteroatom that optimizes the compound for a specific application. Nitrogen heterocycles have been found to mimic various endogenous metabolites and natural products, highlighting their pivotal role in current drug design. Their applications are manifold and are predominantly used as pharmaceuticals, corrosion inhibitors, polymers, agrochemicals, dyes, developers, etc. Additionally, their catalytic behavior has rendered these compounds notable precursors in synthesizing various important organic compounds. The rate at which nitrogen heterocycles are synthesized explains this organic chemistry domain's vitality and usefulness. The present review article focuses on nitrogen-containing heterocycles as a versatile scaffold for current applications of organic chemistry.
1. INTRODUCTION
Heterocyclic chemistry is a major branch of organic chemistry, accounting for nearly half of the known organic compounds. Heterocycles have led to the development of society from a medical as well as industrial point of view. Most pharmaceuticals, dyes, cosmetics, polymers, agrochemicals, etc., are heterocyclic [1-3]. The heterocyclic ring system is of exceptional importance in industry as most heterocycles serve as a basic template for synthesizing various industrially utilizable compounds. Heterocycles are also present in various drugs, natural products, vitamins, biomolecules, dyes, agrochemicals, etc. Some of the heterocycles also possess biochemiluminescence and photochromic properties. Heterocycles exhibit diverse applications in material sciences like analytical reagents, dyes, information storage, sensors, etc. In addition, they are being utilized in polymer chemistry, mainly in conjugated polymers [4-9]. Moreover, they can also act as semiconductors, organic conductors, light-emitting diodes, photovoltaic cells, light-harvesting systems, and liquid crystalline compounds. Heterocycles have proved to be of remarkable interest because of their synthetic utility as catalysts, protecting groups, and metal ligands in inorganic synthesis. Therefore, heterocycles deserve substantial attention, and efficient methods to develop new heterocycles are one of organic chemistry's most prominent work areas. The common heterocycles possess 5 or 6-membered rings containing nitrogen, oxygen, or sulfur. Heterocycles can be classified into aromatic and aliphatic, in which the former comprises cyclic analogs of amides, amines, ethers, etc. Their properties are mainly determined by the strain in the ring. The latter are obtained from aromatic carbocycles with a heteroatom occupying the place of a ring carbon atom. The heteroatom is responsible for various physicochemical properties than that of the precursor carbocycles. The availability of lone pairs of electron/s and the electronegativity difference between heteroatom and carbon considerably impact the nature, reactivity, and structural modifications of heterocycles [10-16]. In this regard, nitrogen heterocycles are of utmost significance as these exhibit diverse biomedical and pharmacological activities and show promising results in varying degrees due to the distinctiveness like substitution, heterocyclic systems, and adjoined pharmacologically active functional groups that make them accessible for the structure-activity relationship studies as well as biological evaluation. The major advantage of such heterocycles is their adjustable nature arising due to compact molecular structures with ample functional diversity. Carbon atoms provide high stability to such rings in accordance with the concept of aromaticity, and the presence of nitrogen gives typical properties to these heterocycles owing to rich electron density. The physiochemical properties of these heterocycles are relevant in the design of various novel materials, particularly organic conductors. Lead identification and derivatization of nitrogen heterocycles with different substituents can lead to their manifold applications [17-21]. The most important ring system related to heterocycles is the five and six-membered compounds containing two or at least one hetero atoms, mainly nitrogen, oxygen or sulfur. Many bioactive compounds contain five-membered nitrogen heterocycles with pyrrolidine moiety. Nicotine, tryptamine and vinblastine are examples of five-membered nitrogen heterocycles with pyrrolidine ring structures that are vital in biochemistry, pharmaceuticals and agriculture. The well-defined conformations of these natural compounds may include 2-pyrrolidine, streptopyrrolidine or diphenylprolinol rings. These ring systems can act as versatile intermediates in synthesizing more complex medicinally important compounds like aniracetam, doxapram, cotinine, clausenamide, lactacystin, detoxime and codonopsinine, in addition to having structural features in many naturally occurring bioactive natural products. Six-membered nitrogen-containing heterocyclic compounds are abundant and are of great significance to life as their structural subunits exist in many natural products such as vitamins, hormones, antibiotics and alkaloids, pharmaceuticals, agrochemicals, dyes and many more compounds [22-27]. This review evaluates potential medicinal and industrial applications of N-bearing heterocyclic compounds (four, five and six-membered rings), including azetidines, thiazoles, thiazines, imidazoles, triazines, tetrazines etc.
2. APPLICATIONS OF NITROGEN-CONTAINING HETEROCYCLES
2.1. Nitrogen Heterocycles as Drugs
Nitrogen-containing heterocycles are amongst the most prominent structural entities of pharmaceuticals. As per U.S FDA pharmaceuticals, a detailed analysis of the N-heterocycles showed that 59% of small-molecule drugs possess nitrogen heterocycles, making them the most significant and privileged structures among heterocycles. The most notable ones incorporated as drugs contain piperazine, morphinan, cepham, penam, or phenothiazine moiety [28-33]. A series of nitrogen heterocycles having distinguishable structural modifications being synthesized possess diverse biological activity (Table 1). Nitrogen heterocycles serve as emerging constituents for drug design as numerous efforts to synthesize nitrogen heterocycles are a field of ongoing research. A series of pyrido-benzothiazine derivatives show prominent antibacterial activity. Among these, the fluoro substituted, namely rufluoxacin, shows in vivo potency on oral administration that is equivalent to ofloxacin and ciprofloxacin. The methyl and pyrido substituted benzothiazines show better absorbance and induced stable plasma and urinary levels. However, the introduction of bulkier groups resulted in a substantial loss of activity. Structural modification of several thiazines, including N-methyl and N-acetyl piperazine derivatives, show prominent anti-fungal activity against P. marfenaffei and A. niger. The incorporation of benzothiazine moiety in the structure of ketoconazole as a biosteric replacement for the 2,4- dichlorophenyl group of ketoconazole was investigated in which the trans analog showed better activity. Benzothiazine derivatives having (1-piperazinyl-4-phenyl) alkyl moiety at 2-position show potent anti-hypertensive activity. As a result, it is possible to develop a new cardiovascular agent that can interact with calcium channels. Amino propyl derivatives of various nitrogen-bearing heterocycles invariably form a new class of anti-inflammatory agents. However, the acetyl derivatives show lesser efficacy than their precursor. Certain methyl and chloro-substituted benzothiazines associated with pyrazine moiety resulted in the formation of potential neuroleptics. Novel methotrexate derivatives were tested for anti-rheumatic activity and claimed to be safe analogs without side effects. Several nitrogen heterocycles also show aldose-reductase activity. For example, 1,4-benzothiazine-2-acetic acid and its bioisosteres showed remarkable inhibition of aldose-reductase in the porcine lens. 4-(substituted benzothiazol)-1,4-benzothiazine-2-acetic acid derivatives show better aldose-reductase inhibitory activity than its chloro or bromo analog. This compound shows aldose-reductase inhibitory activity, resulting in a substantial decrease in sorbitol accumulation in rat sciatic cells. Certain N-heterocycles like 4H-1,4-benzothiazine-2-carbonitrile 1,1-dioxide are found to be active potassium channel openers. Biological evaluation of ribofuranoside derivatives of certain nitrogen heterocycles showed enhanced antioxidant activity than conventional compounds. Hence, it can be considered that ribosylation is primarily responsible for the anti-oxidant property, and the pharmacophore is the heterocyclic ring-bearing sugar moiety [34-52].
Nitrogen Heterocycles | Biological Activity | References |
---|---|---|
![]() |
Anti-bacterial | [53] |
![]() |
Anti-hypertensive | [55] |
![]() |
Anti-inflammatory | [56] |
![]() |
Central nervous activity | [57] |
![]() |
Anti-rheumatic | [58] |
![]() |
Aldose-reductose inhibitor | [59] |
![]() |
Potassium channel opener | [60] |
![]() |
Antioxidant | [61] |
![]() |
Anti-malarial | [62] |
The N-substituted thiadiazole derivatives (Fig. 1) were evaluated for anticancer activity against MCF 7 and A549 cells. The most efficient compound was screened against a non-cancer cell line [54] to determine the specificity of cytotoxic activity. Among the various analyzed compounds, [N-(5-ethyl-1,3,4-thiadiazol-2-yl)-2-((5-(p-tolylamino)-1,3,4-thiadiazol-2-yl)thio]acetamide exhibited enhanced cytotoxic activity against MCF cancer cells [63].
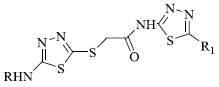
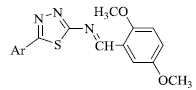
A new class of biomedically prominent thiazoles (Fig. 2) has also been synthesized and tested for anticancer and antimicrobial activities. Some compounds show DNA protection effects against oxidative stress, while a few reported anti-microbial activity against S.epidermidis. Molecular docking analysis was performed using certain selected derivatives, exhibiting drug-like properties for prostate and breast cancers. Considering the minimum inhibitory concentration, the benzofuran derivative of thiazole tends to inhibit the growth of various species of Salmonella and Staphylococcus genus [64].
2.1.1. Rationale of Molecular Design and Structure-Activity Relationship of Certain Anti-Proliferative Compounds
The synthesis of a series of novel pyrimidine derivatives and their reactivity towards various electrophilic and nucleophilic reagents was examined. 3-(4-[dimethylamino] phenyl)-1- (napthalen-2-yl) prop-2-en-1-one after being synthesized via Claisen-Schmidt condensation (Scheme 1) upon subsequent condensation with thiourea, urea and guanidine hydrochloride in basic conditions resulted in the synthesis of 6-(4-[dimethylamino] phenyl)-4-(napthalen-2-yl) pyrimidin-2-(1H)thione, 6-(4-[dimethylamino]phenyl)-4-(napthalen-2-yl) pyrimidin-2 (1H)-one 7 and 4-(4-[dimethylamino]phenyl)-6-(napthalen-2-yl) pyrimidin-2-amine respectively.
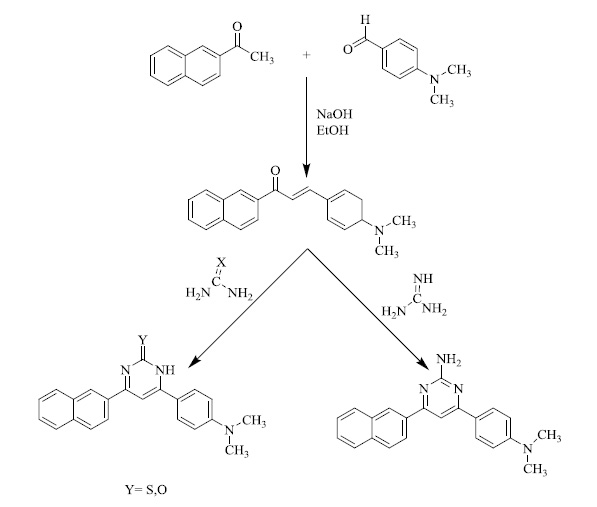
On being treated with benzoyl chloride in the presence of pyridine, pyrimidine derivatives would undergo further derivatization (Scheme 2) to yield various bioequivalent agents.
Also, various derivatives were afforded to treat pyrimidine derivatives with ethyl bromoacetate in DMF in the presence of anhydrous K2CO3. Upon refluxing the same with chloroacetyl chloride using DMF as a solvent and in the presence of anhydrous, potassium carbonate afforded derivatives which after being submitted to methyl 4-(2-chloroacetamide) benzoate compound yielded 2-((4,6-diphenyl-1,2-dihydropyrimidin-2-yl)thio)-N-phenylacetamide derivatives (Scheme. 3) that upon reaction with different amines yields certain bioactive compounds [65, 66].
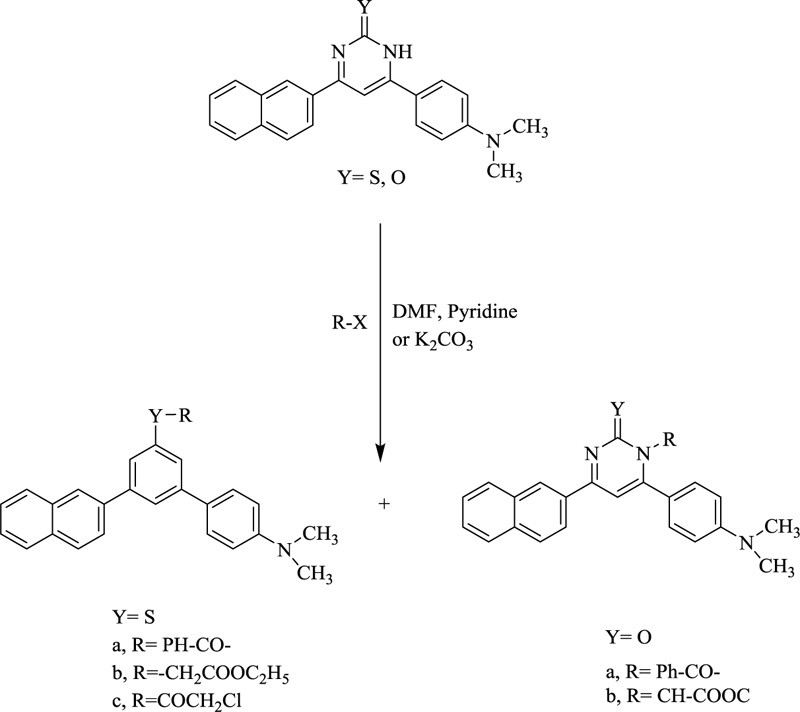
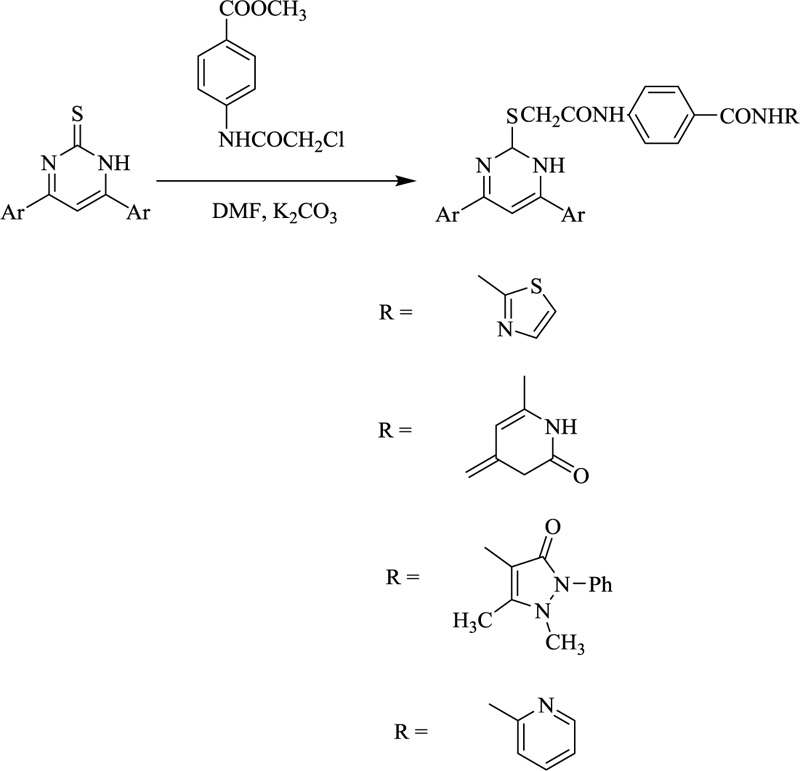
In various similar reactions, compounds like 2-(1-(4-(dimethylamino)phenyl)-3-(naphthalen-2-yl)-3-oxopropyl) cyclohexan-1-one,2-((1-(4-(dimethylamino)phenyl)-3- (naphthalen-2-yl)-3-oxopropyl) thio)acetic acid, 3'-(2-naph thoyl)-4'-(4-(dimethylamino)phenyl)spiro[indoline-3,2'-pyrrolidin]-2-one etc. (Fig. 3) were obtained.
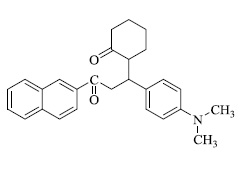
a) 2-(1-(4-(dimethylamino)phenyl)-3-(naphthalen-2-yl)-3-oxopropyl) cyclohexan-1-one
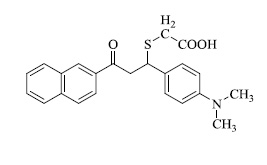
b) 2-((1-(4-(dimethylamino)phenyl)-3-(naphthalen-2-yl)-3-oxopropyl)thio)acetic acid
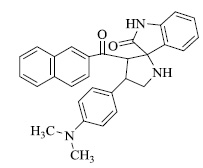
2-(1-(4-(dimethylamino)phenyl)-3-(naphthalen-2-yl)-3-oxopropyl)cyclohexan-1-one, b) 2-((1-(4-(dimethylami no)phenyl)-3-(naphthalen-2-yl)-3-oxopropyl)thio)acetic acid, c) 3'-(2-naphthoyl)-4'-(4-(dimethylamino)phenyl)spiro[indoline -3,2'-pyrrolidin]-2-one (Fig. 3a).
All the synthesized products were screened for anticancer activity against MCF-7 and HePG-2 cell lines. Many of these exhibit diverse pharmacological properties. A molecular docking study has also revealed their interaction with dihydrofolate reductase (DHFR) active sites. An overview of the structure-activity relationships(SAR) (Fig. 4) revealed that DHFR antagonists have four significant features:

- A heteroaromatic ring system with at least one H-bond acceptor and one H-bond donor.
- A linker moiety
- A spacer which comprises an aryl ring
- Terminal pharmacophore consists of at least two hydrogen bond acceptors.
The basic core of the molecular design rationale comprises bio-isosteric moderation strategies of DHFR inhibitors at four distinct positions.
By comparing the experimental cytotoxicity of various pyrimidine derivatives, the following structure-activity relationships (SAR) were suggested.
- The linker region bearing sulfur atoms has maximum cytotoxic activity. (IC50±2µM)
- Introducing COCH2Cl to the pyrimidine moiety and the sulfur atom enhances cytotoxic activity. (IC50=9.17±1.0µM)
- Including thioacetic acid in the linker also adds to the cytotoxic activity. (IC50=19.42±1.9µM)
- The spiro derivative has a higher cytotoxic effect than the subsequent derivatives.
Furthermore, molecular docking of the active site of the dihydrofolate reductase (DHFR) enzyme with the pyrimidine derivatives was conducted to investigate their binding interactions/modes. Additionally, scrutinizing the binding pattern between the DHFR enzyme and the most active compound and determination of the interaction energy reveals that it involves the formation of π-bonds [67].
2.2. Nitrogen Heterocycles as Polymers
The ability of nitrogen heterocycles to bond with metals, act as ligands, to undergo hydrogen bonding with proteins and drugs has resulted in their utility as multifunctional polymers. These polymers have diverse applications. For example, hydrogels obtained via polymerized methylimidazolium-based ionic liquids (Fig. 5) have prominent bioactive applications as these polymers self-assemble into arranged lamella with distinct swelling properties. Nitrogen heterocycles afford many biophysical applications, and their polymers represent a new and comparatively unexplored field. The supra-molecular association of these biopolymers for various antimycotic and antimicrobial drug applications has recently been of keen interest [68].
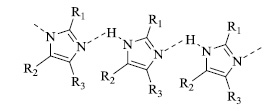
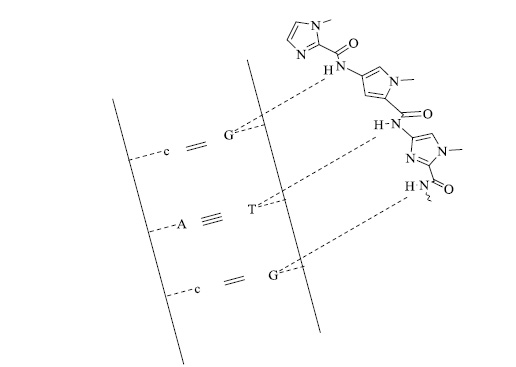
Certain nitrogen heterocycles, like imidazoles, act as precursors for various polymers. For example, vinyl-substituted imidazoles tend to bond with cobalt oxygen carriers and form reversible oxygen-binding polymer membranes for oxygen transport. Many other bioactive nitrogen heterocycles also form a distinct class of polymers. N-methyl pyrrole-containing heterocycles (Fig. 6) have also been synthesized for sequence-specific DNA alkylation [69].
The symmetrical azine-based polymers, after being synthesized, were evaluated for photoelectrical, optical, and thermal properties. Nitrogen Heterocycles also constitute one of the major classes of electroactive polymers. When exposed to a specific electrolyte solution, these materials exhibit ionic and electronic activity. Low molecular weight piperazine-based film-forming cationic polymer is used as a cosmetic hair conditioner in amounts of about 0.1-5 weight percent of the composition in solvents like alcohol or water. Nitrogen heterocycles like polypyrroles have proved to be very promising materials in forming miniature electrochemical immunosensors [70]. Polypyrroles are biocompatible and cause reversible and minimal disturbance to the functioning environment. These are mostly biosensors because of their compatibility and ease of immobilization. Polypyrrole-based stimulus-responsive biopolymers are favorable starting materials for immunosensors, preferably for various biological applications. These are also being used in chemical sensor technology as molecularly imprinted polymers. These possess molecular recognition property that arises as a result of cavities in the polymer matrix that are complementary to the analyte in positioning and shape of the functional groups. Many of these polymers also show high-affinity constants that can be compared to naturally occurring recognition systems like antibodies, making them desired compounds for immunosensors. Polypyrroles are imprinted by neutral and charged molecules in certain affinity sensors. Such application of conducting polymers in association with bio-affinity reagents has put forward a desirable route to extend their use in electrochemical detection. Furthermore, polyazines containing polypyrrole moieties are considered high-performance ambipolar semiconductors in organic thin-film transistors [71]. Polyazines are well-known polymer substances arising as a prominent division of semiconductor materials that can be used as organic thin film transistors. These are formed via azine linkage formation like a condensation of a π-conjugated diketone or dialdehyde compound with hydrazine without incorporating any catalyst under mild conditions. Polyazines display a characteristic ambipolar behaviour, which finds application in logic circuits. Including the azine linkage to design other conjugated polymers and their use in organic electronics like organic photovoltaics is also gaining interest in the polymer industry. The main advantage of polyazines as polymers lies in their environmental stability, architectural flexibility, solubility, and high optical absorption. Polyazoles are significantly used in synthesizing polymer membranes and films, which possess improved mechanical properties and retention of polymer membranes above the operating temperature of 100ºC. The acid-doped polymer membranes based on polyazoles have diverse applications in fuel cells, battery systems, capacitors, etc. Polyazoles like polyoxadiozole and polytriazole are used under membrane technology in contact membrane distillation operation [72-74]. Polypyridine complexes are used in the synthesis of luminescent transition-metal complexes, and notably, these complexes have cellular and biological applications with a focus on their use as phototherapeutic agents and bioimaging reagents, biomolecular probes because of their rich photochemical and photophysical properties. Target delivery is specific to malignant cells only. Organic photovoltaics have shown an exponential increase in production due to the introduction of low-cost organic semiconductors. Recent research suggests the use of azine-based π-conjugated polymers, which are promising semiconductors for electronics. In this regard, a new azine-based polymer, PDDBTA (Fig. 7), has already been synthesized, which acts as a donor in organic photovoltaics and a p-type semiconductor channel in organic thin-film transistors. The preliminary results have further enhanced the usage of azine-based π-conjugated polymers as a favorable distinct class of cost-friendly organic semiconductors and other printed electronics [75-80].
DFT simulation results have shown that the inclusion of azine in the bithiophene azine subunit has lower LUMO and HOMO than bithiophene, making it desirable for use in organic photovoltaics. N-based heterocycles constitute an important class of energetic materials as these can store a high amount of energy that could be derived by decomposition and the formation of gaseous by-products. These energetic materials are designed to fulfill the arising need for propellants and payloads. The amount of energy stored in a compound is directly associated with the chemical composition. By linking the proper backbone with the desired functional groups, the energetic characteristics of the compounds can be altered and refined. High nitrogen compounds that procure their energy from the intrinsic high heat of formation have gained interest in modern research. Along with their high thermal stability, nitrogen-containing heterocycles like triazines, tetrazines, triazoles, tetrazoles provide better backbones to produce new energetic compounds like 3,3' azobis(6-amino)-1,2,4,5-tetrazine, 4,4',6,6',tetra(azido)azo-1,3,5-triazine, (Fig. 8) etc. These heterocycles are further modified by introducing functional groups like N3, NH2, etc., which also increases the overall nitrogen content and heat of formation [81].
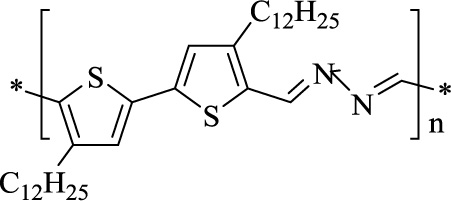
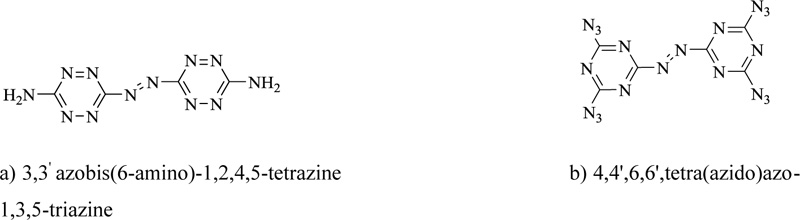
a) 3,3' azobis(6-amino)-1,2,4,5-tetrazine b) 4,4',6,6',tetra (azido)azo-1, 3,5-triazine
C-azo linkages should also possess an N,N-azo linkage along with a long chain of catenated nitrogen atoms that further enhances the nitrogen content, eventually increasing the energy as well as the density of the compound to obtain stable energetic high nitrogen compounds. These compounds form the basic structure that can be modulated due to the tenability of the ring carbon atoms. A series of polyazido and polyamino substituted high-energy nitrogen compounds have already been characterized. The physical and chemical characteristics of the synthesized products were also obtained. 3,3',5,5' - tetra(azido)azo-1,2,4-triazole was found to have very high heat of formation and a relatively higher decomposition temperature. The high stability and ease of reactions of N, N’- azo heteroatomic compounds make them potential precursors for various desired modifications giving rise to diverse high-nitrogen compounds [82, 83].
2.3. Nitrogen Heterocycles as Agrochemicals
Nitrogen heterocycles have arisen as one of the prominent agents for crop protection. Cutting-edge development in the synthesis and design of agrochemicals has formed the backbone of the agrochemical industry. More than 70% of the already introduced agrochemicals possess heterocyclic scaffolds primarily containing one or more nitrogen atoms. State-of-the-art organic chemistry used to manufacture advanced crop protection chemicals includes the production of three commercially available fungicides, boscalid, fludioxonil, and benomyl [84]. Crop protection chemistry has occupied an important domain in organic chemistry as structure-based designs are needed to achieve the required targets. Pinoxaden is a new herbicide used to reduce the growth of grass weeds in cereals. Similarly, the nitrogen-containing fungicide Bixafen shows significant activity against a broad spectrum of cereal diseases. Another commonly used insecticide is a nitrogen heterocycle called Indoxacarb (Fig. 9), which is effective against Lepidoptera in fruits, vegetables, and cotton [85].
a) Pinoxaden b) Bixafen c) Indoxacarb
The structure-based design has been an emerging discipline within crop protection research. The likelihood of finding better analogs has increased by incorporating virtual screening methods. Nitrogen heterocycles form prominent scaffolds in this regard. A potential library of such herbicides can be generated, keeping the core structure the same and altering the attached linkers. Structural optimization of certain basic nitrogen heterocycles has led to the discovery of various novel heterocycles. For example, using heterocyclic herbicides like flupropacil and imadapyracil as a template led to the discovery of triazoylpyridines possessing fungicidal activity from the former, and phenylpyridadinones were selected as leads from the latter. Better analogs were synthesized after replacing the 1,3,5-triazine nucleus with a pyrimidine nucleus, considering the structural similarity and the characteristics of known bleaching herbicides. A distinct class of fungicides has arisen due to speculative chemistry that attempted to introduce the carboxylic group into the pyridine nucleus. N-butylated pyridines have also shown dominant fungicidal properties. This compound became a lead in synthesizing many potent fungicides that have shown better results and a broad fungicidal spectrum. This led to the discovery of the fact that the 2,6-disubstituted pyridine ring is one of the major structures that possess fungicidal activity. Dramatically enhanced fungicidal activity has been reported in derivatives comprising a 1,2,4-triazole ring attached to the pyridine ring. Structure-activity relationship studies reveal that a striking change in the activity can be observed by replacing the pyrimidine ring with a pyridine ring. Finally, pyridinyl pyrimidine derivatives were selected as promising candidates for pre-commercialization. Moreover, introducing certain groups like the triflouromethyl group at position 6 of the pyrimidindione ring significantly added to its herbicidal activity. Upon structural optimization of flupropacil, the herbicidal activity was noticed while changing the heterocyclic ring, the phenyl ring or the substituents, and it was revealed that the 2,4,5-trisubstituted phenyl ring would lead to optimum activity. Nitrogen heterocycles have also proved beneficial in controlling undesired vegetation, unchecked growth of weeds, a significant reduction in productivity, etc. The invention of certain heteroaryl azoles and their N-oxides is noteworthy [86, 87]. Certain heterocyclic compounds like azines or azoles bearing trifluoromethoxy group show remarkable biological activity and are used as pro-insecticides, growth regulators, and herbicides. In this context, 2-amino-5-trifluromethoxy benzothiazole and thiazolo-5-carboxylic acid are known for their fungicidal property. 2-thiohydantoins are also being utilized in the synthesis of various fungicides and herbicides. The incorporation of the trifluoromethoxy group further enhances its activity in the structure. The group also showed a similar effect on being linked to pyrimidines and quinolines. Certain azoles are also responsible for enhancing the resistance of plants toward various abiotic factors. The fungicidal action of various azoles like prothioconazole and tebuconazole is very well known and is based on sterol biosynthesis inhibition. These stress-tolerating effects are expected to give a linear correlation of stunting or growth regulatory action. In oilseed, it has been observed that a combination of certain azoles with abscisic acid increases the plant resistance against certain abiotic factors that result in abnormal stunting of plants. It has been revealed that fertilizers that usually counteract the abiotic stress conditions like osmotic stress, water-logging, drought, cold temperature, heat exposure, salinity, increased exposure to minerals, etc., are mostly nitrogen-bearing heterocycles. In this regard, mention should be made of NPK fertilizers that are highly commercialized. Insecticidal compounds of general formula (Fig. 10);
Where A represents a 6/5 membered heteroaryl group containing one or more heteroatoms selected from N, O, or S. Z represents a three-membered straight chain with at least one of the three members being CH2-, O, S or N-R′ where R′ represents the group (Fig. 11);
Where R”represents hydrogen or halogen atom, X represents CH or N, R represents a methyl group or hydrogen atom, and Y represents nitro or cyanogroup [88]. A series of sulfanyl derivatives of thiadiazoles have been synthesized and characterized by spectral and analytical data as it is a significant biological scaffold. The bioefficacy of these products was evaluated against two phytopathogenic fungi, Rhizoctonia solani and Rhizoctonia bataticola. The most effective compounds among these were nanosized to further enhance their fungicidal activity. The nano-forms of 1,3,4-thiadiazole derivatives were characterized via electron microscopy and a particle size analyzer. The nano-forms showed 2-3 times higher fungicidal activity than the corresponding conventional-sized 1,3,4-thiadiazole derivatives. Nano sizing of potent thiadiazoles thus enhanced the bioactivity of those heterocycles more than the generally used compounds. Certain novel heterocycles have shown predominant insecticidal activity. Arylalkylimidazole (Fig. 12) shows prominent insecticidal activity against cotton aphids.
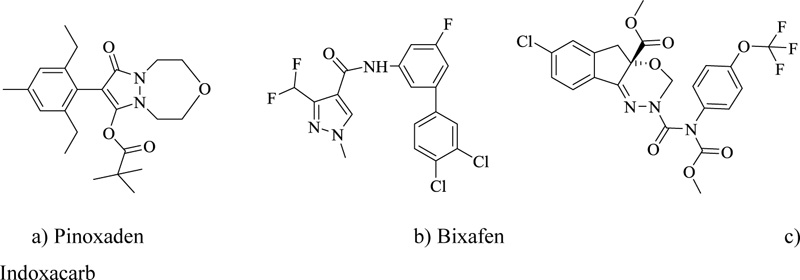
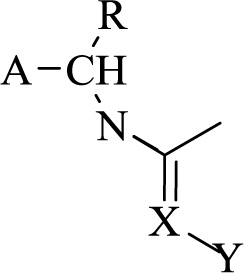
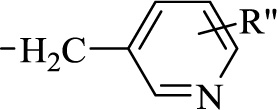
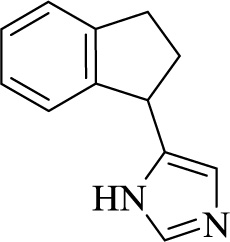
In this regard, certain heterocyclic compounds are active ingredients in insecticidal composition [89].
2.4. Nitrogen Heterocycles as Dyes
Heterocyclic compounds as dyes are still being utilized in traditional protocols, but these are also key constituents in fluorescent probes. These dyes show a promising paradigm shift in technology and are being implemented in solar cell technology, optoelectronics, photodynamic therapy, and photoinduced electron transfer imaging (PET). Nitrogen-bearing heterocycle dyes have varied applications in diverse fields like analytic chemistry, pharmaceuticals, textile, cosmetics, and leather. These are, so far, the most important class of dyes, accounting for over 50% of the total annual production. Dyestuff chemistry has attained a distinct place in synthetic organic chemistry, considering the relevance of colorants in modern times. Modern industrial organic chemistry initiated the growth and development of synthetic dyes. Heterocyclic compounds are being utilized as synthetic and naturally occurring food dyes, colorants in inkjet printing, photography, and are predominantly used in commercially significant textile dyes. The field of dyestuff chemistry is extensive, and the introduction of nitrogen heterocycles has further revolutionized the domain [90]. Pyrroloids like Basic Yellow have been extensively used in paper dyeing, inkjet printing, textile printing, and dyeing, largely in optical data storage. The benzo analogs of indolenes are also known for their effect of dye structural elements on fluorescent properties. The benzoindolium derivatives generate a variety of visible and NIR dyes that are already commercialized. Symmetrical indocyanines have been used as well-known dyes for a long. The dyes possessing unsymmetrical structures having two distinct heterocycles are of keen interest as they are used as chemo-sensors because of their high sensitivity towards external factors. Moreover, these dyes' structural modifications allow fine-tuning of several chemical and physical features, including fluorescence parameters and solubility. The commonly employed synthetic procedure involves the stepwise addition reaction between a proper polyene precursor and an indolium salt. In association with various cyanine dyes, this chromophore showed a power conversion efficiency of about 3.0% in TiO2 dye-sensitized nanocrystalline solar cells. The polymethine group incorporated between indolenes and other heterocyclic groups has various characteristics. For example, the pyrimidine-fused benzoindolenes is a well-known pH-sensitive NIR fluorescent probe with a pKa of 3.5. The hemicyanine-coumarin hybrid developed is a useful far-red emitting fluorogenic probe for penicillin G acylase (PGA). The highly stable squarylium moiety provides some useful and interesting properties to chromophores. For example, novel-water soluble symmetrical squarylium dyes with benzyl N-substituents inhibit aggregation and increase photostability in water and fluorescence. Another important class of pyranoid dyes comprises carbazoles. These have recently joined the ranks of designer dyes and are also being evaluated for use in dye-sensitized solar cells. For example, Indathren Olive R is a long-established textile dye currently used for military camouflage fabrics as it can color synthetic materials that correspond to the reflectance profile of leaves in the NIR region. C2 symmetric3,6-disubstituted carbazole-based bisboronic acid acts as an excellent enantioselective fluorescent sensor for various organic acids such as tartaric acid. Profoundly, the fluorescence is enhanced at a higher pH. These are also being employed in the synthesis of polymeric arrays. The boron dipyrromethene dyes have gained keen impetus in recent years and have come to light due to their own bioanalytically related fluorophores because of their large quantum yields and relatively higher extinction coefficients. Another interesting development in pyrroloid dyes is using phenolic dipyrrole as a chromogenic probe for radiometric determination of water in organic solvents (Fig. 13). Unsymmetrical bipyrroles act as an electrochemical dye as these can induce color changes at low potential. In solution, the dipyrroloazo dye can distinguish calorimetrically among fluoride, acetate, and dihydrogen phosphate anions. The diketopyrrolopyrroles are high-performance pigments primarily used in paint formulations, plastics, and dye-sensitized solar cells. Diketopyrrolopyrroles, associated with cyanine, are being designed for near IR dyes and fluorophores [91].
a) N,N'-(5,9,14,17-tetraoxo-9,14,16,17-tetrahydro-5H-dinaphtho[2,3-a:2',3'-h]carbazole-6,8-diyl)dibenzamide
b) (E)-2-(2-((2,4-dimethoxyphenyl)amino)vinyl)-1,3,3-trimethyl-3H-indol-1-ium
c) Substituted (E)-2-((2-hydroxy-3-((1-methylindolin-2-yl)methyl)-4-oxocyclobut-2-en-1-ylidene)methyl)-1,3,3-trimethyl-3H-indol-1-ium
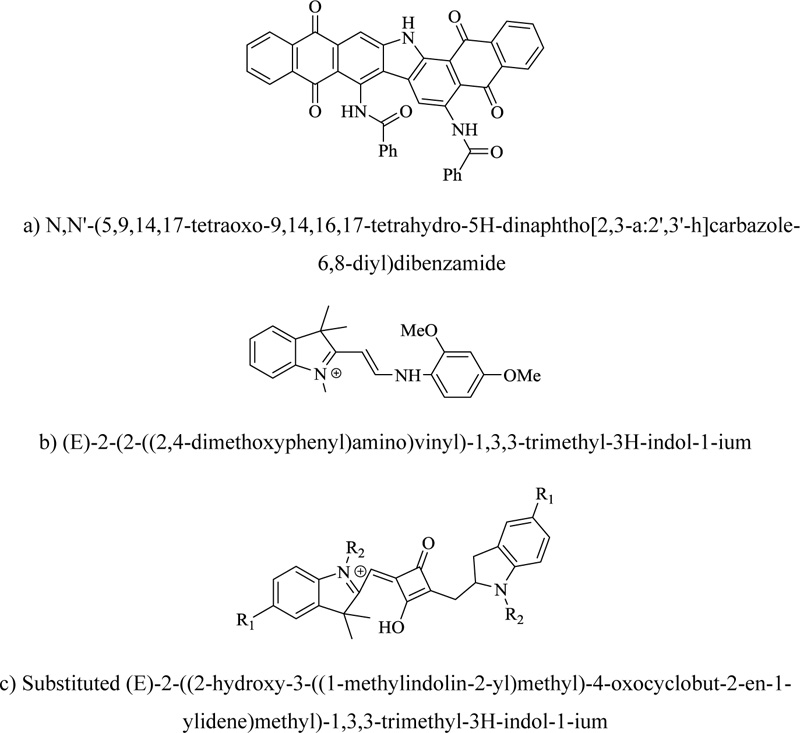
Imidazole-containing hetero-system is present in traditionally used dyes and many fluorescent whitening agents. Moreover, various prominent innovations containing imidazole derivatives have also been characterized. For example, a reactive UV-absorber like cyanuric chloride in association with benzimidazole has been developed to create UV-opaque textile materials. Moreover, sulfonated benzimidazole is one of the main conventional components in dye chemistry. A turn-on fluorescent probe for cyanide has been designed with an acceptor-donor-acceptor sensor. In such sensors, imidazole acts as a donor. Certain imidazole-bearing novel heterocycles (Fig. 14) exhibit interesting solvatochromic properties. Similar compounds also show sensitive behavior towards homocysteine and cysteine. Certain imidazoles like hexaarylbiimidazole show the practical photochromic utility and are known for undergoing photolytic cleavage. Photochromism is also observed in heterocycles like 1,3-diazabicyclo [3.1.0] hexanes [92].
a) (4-(1-methyl-4,9-dioxo-3a,4,9,9a-tetrahydro-1H-naphtho[2,3-d]imidazol-2-yl)phenyl)boronic acid
b) Dimethyl 4,4'-((1E,1'E)-(2-(4-formylphenyl)-1H-imidazole-4,5-diyl)bis(ethene-2,1-diyl))dibenzoate
Six-membered heterocyclic rings containing nitrogen are also known for their significant dye-inducing ability. One of the major groups is pyridinoid scaffold-bearing dyes like Disperse Yellow, which is used in textiles, optical storage, and neon art materials. This structure provides a basic template for functional elaboration. Another dye, namely dye 190, also possesses a similar structural framework and is a highly photostable on-off pH switcher and metal ion selective fluorescent probe. Napthalenebisimide dyes are reported to possess excimer emission. This behavior is highly selective for pyrophosphate in the presence of phosphate and ATP, making it an application for further exploration. Another class of dyes, perylene, like Pigment Red 179, are of significant commercial importance as these are high-performance colorants. In addition, perylenes are also being used in several other innovative products. Substituted perylenes are also used as potential building blocks in photovoltaic devices. Disulfonated perylene is applied in inkjet printer liquid crystal display colour filters. Z-shaped perylene bisimide is a fluorescent dye that dissolves in polar organic solvents and can be incorporated into polystyrene films. The fluorescence emission is 517nm, with a quantum yield of about 0.67. Some of these dyes (Fig. 15) have interesting optical properties. A series of such compounds called rainbow perylene monoimides have potential application in solid-state chemistry due to their absorption and electrochemical properties tuning ability. Pyridine ring finds a place in various chromogenically interesting architectures [93].
a) 2,9-dimethylanthra[2,1,9-def:6,5,10-d'e'f']diisoquinoline-1,3,8,10(2H,9H)-tetraone
b) 6-amino-2-(2,4-dimethylphenyl)-1H-benzo[de]isoquinoline-1,3(2H)-dione
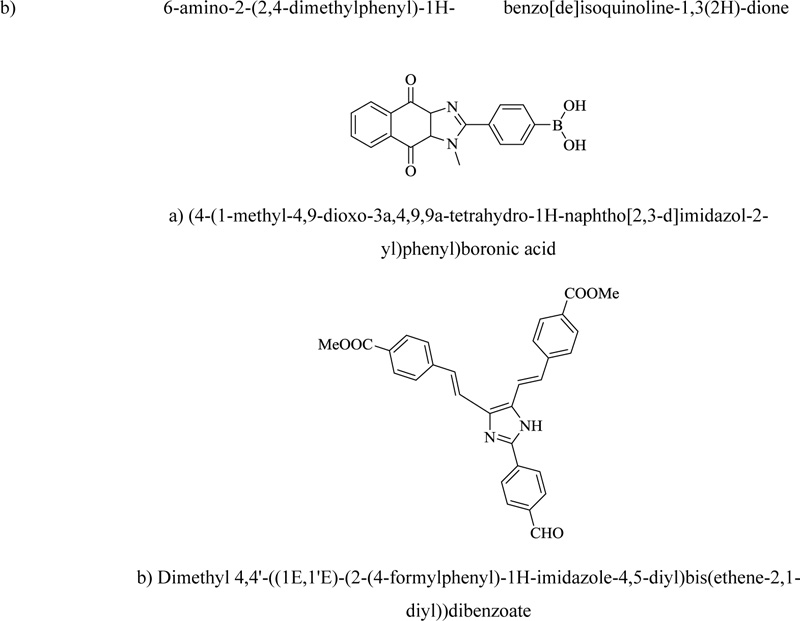
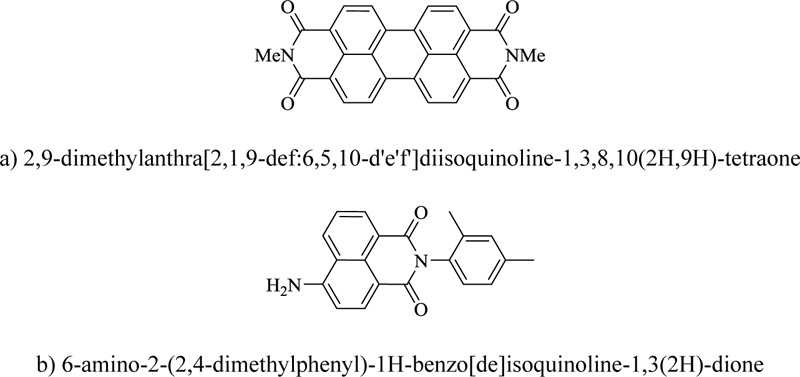
The pyrazine ring is an easily accessible heterocyclic template and a convenient moiety for functionally diverse chromophores. For example, quinoxaline is a useful structure for designing electron and hole-transporting elements for organic light-emitting diodes. A panchromatic series of fluorophores are also synthesized by modification of electronic features at different positions of the heterocycle. A series of the low band gap, film-forming chromophores are designed via molecular modeling to be near IR-absorbing fluorophores. Various derivatives of quinoxaline (Fig. 16) have also been synthesized for being used as polymerization sensitizers in the visible wavelength. Similarly, the condensation between phenylenediamine and cinnamil provided bis (phenylvinyl) quinoxaline, which has a fluorescence emission maximum at 449 nm [94].
a) 4',4”'-(6-(4-(diphenylamino)phenyl)quinoxaline-2,3-diyl)bis(N,N-diphenyl-[1,1'-biphenyl]-4-amine)
b) 4,4'-(2,3-diphenylquinoxaline-5,8-diyl)bis(N,N-dimethylaniline)
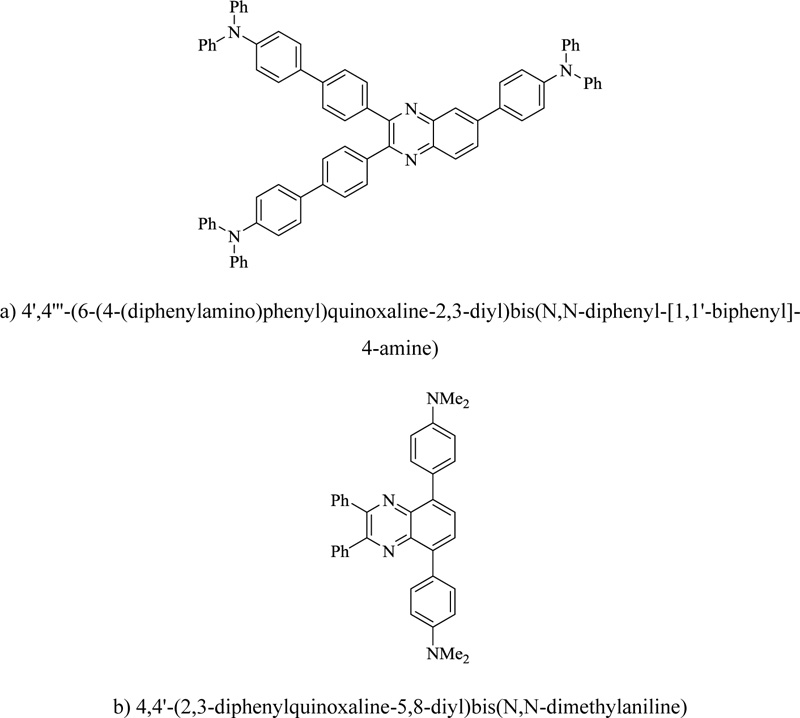
Thermal isomerization kinetics has been reported in neutral azo dyes like those in benzothiazoles. These green light activable molecules have become promising building blocks for molecular switches.
2.5. Nitrogen Heterocycles as Corrosion Inhibitors
Iron and its alloys are essential and are being used extensively in industries. As such, it becomes mandatory to identify materials for their corrosion inhibition. Corrosion inhibitors ensure that there is no/less metal dissolution. In this regard, it has been observed that organic compounds that possess electronegative functional groups and conjugated π electrons exhibit good inhibitive properties. There is also a peculiar interaction between the metal surface and functional groups that contain heteroatoms like nitrogen, oxygen, and sulfur. Nitrogen heterocycles like 4-chloro-1H-pyrazolo[3,4-d] pyrimidine in 1M HCl revealed good anticorrosion activity for mild steel. Inhibition activity, in this case, increases with an increase in concentration and decreases with a temperature rise. Inhibition is the type of mixed-type inhibitor with more anodic effects. Various methods like galvanostatic polarization, hydrogen evolution, potentiodynamic anodic polarization, and electrochemical impedance reveal 2,6-bis-[1-(2-phenylhydrazono)ethyl]pyridine show significant corrosion inhibition for zinc electrode in 1.0M HCl. Another compound showing similar activity would be acenaptho[1,2-b] pyrazine which acts as a corrosion inhibitor in 1M H2SO4. Results show the inhibition activity increase with an increase in inhibition activity. Nitrogen heterocycles, particularly pyrazine and quinoxaline derivatives, are common corrosion inhibitors (Table 2) mainly due to the presence of two or more nitrogen atoms that facilitates electrophilic attack. Many quinoxaline derivatives are currently being explored for their significant corrosion inhibition activity against copper and steel in an acidic medium. Similarly, pyrazines are a component of certain polycyclic compounds of industrial and biomedical importance. All its compounds can act as bridging ligands and have a characteristic low-lying unoccupied π-molecular orbital. Due to this, pyrazines have been preferably investigated as corrosion inhibitors in different acidic mediums. Pyrazines and their derivatives have different inhibition activities depending on the nature of metal-heteroatom interactions of any specific metal in an acidic solution and the presence of a lone pair of electrons on heteroatoms. Recently, molecular simulation studies have revealed the mechanism of corrosion inhibition via the interaction of molecules with the surface atoms of the metal. Electrochemical Impedance spectroscopy gives a detailed analysis of the kinetics of surface properties and electrode processes [95]. Augmentin, also a nitrogen heterocycle, showed maximum inhibition efficiency of 93.6% at 300 ppm and behaved as a mixed-type inhibitor. Lansoprazole and Esomeprazole showed prominent corrosion inhibition properties for copper in 1M HNO3 revealed by using Tafel polarization, electrochemical impedance spectroscopy, scanning electron microscopy, and weight loss techniques. Adsorption was physisorption and fitted with Langmuir adsorption isotherm [96]. Amlodipine Besylate showed corrosion inhibition activity that increases with an increase in concentration. Nifedipine investigation on corrosion inhibition shows cathodic dominance for corrosion of mild steel in 1M HCl. Benzothiazole shows corrosion inhibition efficiency on stainless steel in 3M H2SO4 after being analyzed via various techniques like potentiodynamic polarization, optical microscopy, IR spectroscopy, etc. Results revealed effective corrosion inhibition and significant chemisorption. Cephapirin acts as a corrosion inhibitor for carbon steel in HCl and behaves like a good mixed-type inhibitor. Another Nitrogen heterocycle employed for corrosion inhibition is Clopidogrel, and the efficiency reached 80-90%. Adsorption was both physical and chemical [97-107].
Structure | Name | References |
---|---|---|
![]() |
4-chloro-1H-pyrazolo[3,4-d]pyrimidine | [95] |
![]() |
Esomeprazole | [96] |
![]() |
Lansoprazole | [97] |
![]() |
2,6-bis-[1-(2-phenylhydrazono)ethyl]pyridine | [98] |
![]() |
Acenaphtho[1,2-b]pyrazine | [99] |
![]() |
Augmentin | [100] |
![]() |
Benzothiazole | [101] |
![]() |
Cephaphirin | [102] |
![]() |
Linezolid | [103] |
![]() |
Nifedipine | [105] |
![]() |
Clopidogrel | [106] |
![]() |
4-Amino-3-butyl-5-mercapto-1,2,4-triazole | [107] |
Certain thiazines have been reported to possess prominent anticorrosion activity. The inhibition activity of 2-(4-chlorophenyl)-1,4-benzothiazin-3-one has been evaluated for corrosion control of mild steel in 2M phosphoric acid and employing various techniques like weight loss measurement, electrochemical impedance spectroscopy, and potentiodynamic polarization [103-107].
2.6. Nitrogen Heterocycles as Catalysts
Various transition metal complexes with N-heterocyclic carbene (NHC) ligands have been used in catalytic research and cover a broad domain of reactions ranging from olefinic polymerization to C-C coupling. NHCs are neutral, two-electron-giving ligands with a heterocyclic moiety mainly comprising pyrrazole, triazole, tetrazole, benzimidazole, and imidazole. These can chelate with both hard and soft acids. They constitute a prominent class of N-heterocycles characterized by three or two nitrogen atoms in the precursor compound. A major contributing factor to the emergence of metal NHC chemistry is the enhanced, detailed insight into the nature of central/metal ion, the bonding, the type of heterosystem, and their catalytic behavior [108]. Although Wanzlick and Öfele independently investigated transition metal complexes with N-heterocyclic carbene (NHC) ligands in the late 1960s, these fascinating species remained unexplored for many years. In 1991, Arduengo and colleagues produced the first stable and crystalline N-heterocyclic carbene (IAd) [109]. NHCs have been widely employed as organ catalysts and ligands for several transition metals catalysed processes, owing to the ease with which their steric and electrical properties may be fine-tuned [110]. Olefin metathesis [111-114] is a valuable technique for forming numerous carbon-carbon double bonds. Anderson and Merckling (Du Pont–norbornene polymerization), Banks and Bailey (Philips Petroleum—so-called the three-olefin process) [115], and Natta (linear and cyclic olefin polymerization) [116] were among the first to investigate this reaction. Undefined catalytic systems and severe circumstances were frequently used in these early contributions, limiting the applicability of this transition to relatively simple systems. The discovery of Schrock's molybdenum [117] and Grubbs' first-generation ruthenium [118] complexes in the 1990s significantly increased the applicability of this methodology, but it was only after the introduction of the so-called second-generation Ru catalysts, i.e., Ru-complexes bearing at least one NHC ligand, that the real avalanche of olefin metathesis applications began. Several complexes are currently commercially available, including general-use catalysts such as Umicore Grubbs Catalyst M2a (1a) introduced in 1999 and its SIPr variant (1b], Umicore M2 (2a), Hoveyda–Grubbs' catalyst (3a) and its SIPr analogue, and nitro-catalysts 4a, b (Fig. 17) [119-122].
2.6.1. Ru-NHC Complexes
Ruthenium-catalyzed olefin metathesis is one of the most important and well-studied reactions mediated by NHC–metal complexes. The use of NHC-stabilized ruthenium complexes (second-generation catalysts) has accelerated the development of this approach, which is now considered a must-have synthetic tool for both organic and polymer chemists. Indeed, using NHCs as ligands for ruthenium olefin metathesis has opened hitherto unexplored reactivity routes and permitted significant progress in various difficult reactions [123-125]. Manipulating the NHC scaffold of commercially available second-generation catalysts is at the heart of most efforts to build novel ruthenium catalysts. Moreover, fine-tuning the steric and electronic characteristics of the NHC ligand can significantly impact the catalytic behaviour of the following ruthenium complexes. Unsymmetrical NHCs (uNHCs), which can distinguish steric bulkiness in the vicinity of the carbenic core, have significantly influenced the reactivity and selectivity of the resultant catalysts. Among the several types of ruthenium catalysts that produce uNHCs, those with N-alkyl/N-aryl substituents have gotten much attention. The Grubbs group (Fig. 18) introduced a series of extremely Z-selective ruthenium catalysts with a bidentate unsymmetrical NHC ligand, which was a big development in this sector [126].
2.6.1.1. Ruthenium carbene complexes bearing two unsaturated imidazolin-2-ylidene ligands
Hermann and co-workers described the first examples of ruthenium-based compounds in which both phosphines are substituted by more Lewis basic imidazolin-2- ylidene groups. [RuCl2(PR3)2(CHPh)] is a phosphane compound that interacts with the appropriate imidazolin-2-ylidene to form the air-stable solids 1 and 2 (Cy = cyclohexyl, Naph = 1-naphthyl) (Fig. 19) [127].
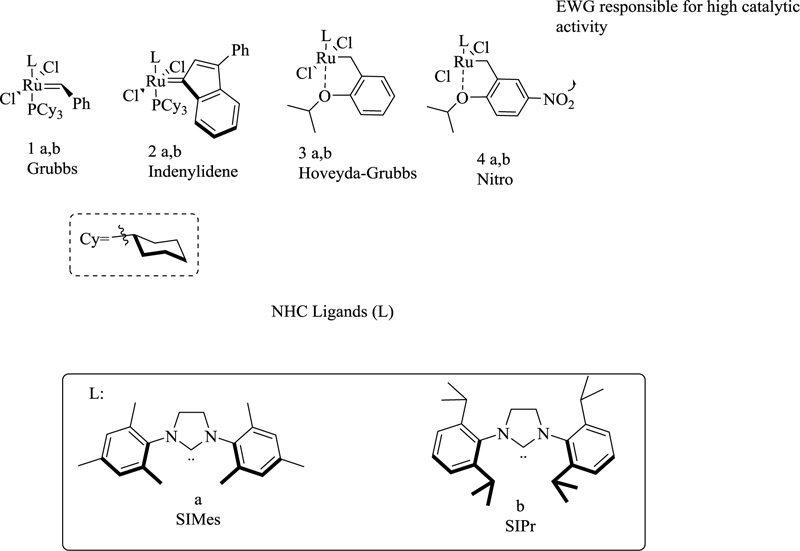
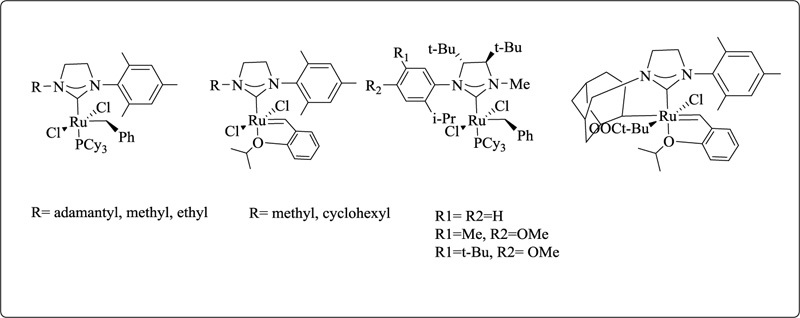
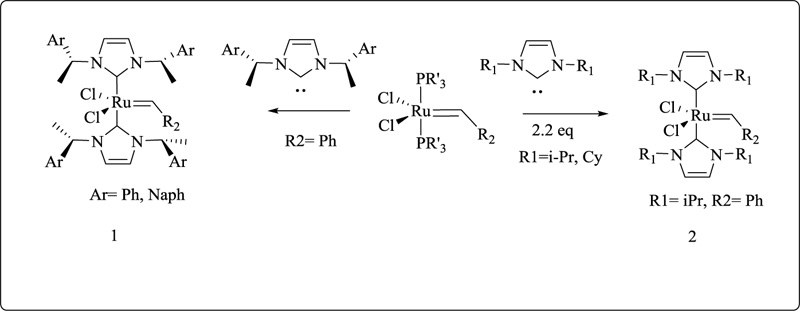
Compounds 1-2 have a high tolerance for functional groups and are very active catalysts in ring-opening metathesis polymerization (ROMP) and ring-closing metathesis (RCM) of any kind.
2.6.1.2. Homo- and Heterobimetallic Ruthenium Alkylidene Complexes
The first example of bimetallic ruthenium NHC complex was prepared by Herrmann and colleagues [128]. (Fig. 20).
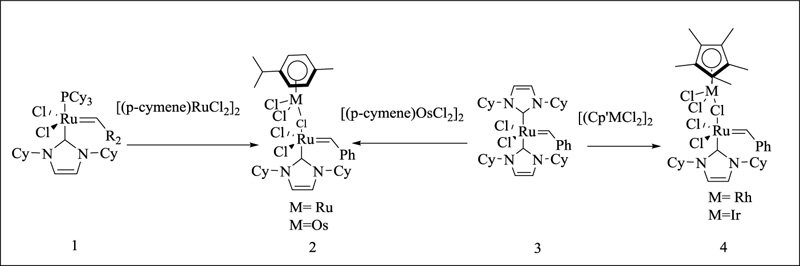
The attraction of distinct metal pieces to NHCs is revealed by differences in the reactivity of chloro-bridged organometallic precursors. Compound 2 can only be made from 1 and [(p-cymene) RuCl2]2, which substitutes the phosphane ligand of 1 selectively while leaving NHC unaltered. In contrast, starting from 1 results in a mixture of heterobimetallic phosphane and NHC complexes; starting from 3 results in a mixture of heterobimetallic phosphane and NHC complexes. The differing affinity of the NHC for chloro-bridged compounds is the deciding factor in reactivity: Rh(III) > Ir(III) > Os(II) > Ru(II) (no reaction).
2.6.2. Cu-NHC Complexes
Though copper chemistry has a long history, the discovery of N-heterocyclic carbene (NHC) as a transition-metal supportive ligand only a few years ago has opened up new vistas in [copper reactivity and catalysis [129]. Raubenheimer published a neutral copper carbene complex shortly after Arduengo's major finding. The field, however, remained inert for nearly ten years. New advancements were made in the early 2000s: first, Danopoulos reported the synthesis of NHC-copper using Cu2O, followed by Woodward's initial application in catalysis [130]. The following paper was by Buchwald and Sadighi, who described the first catalysis utilising a well-defined complex. The early findings in this subject were based on systems designed to imitate their phosphine cousins. Because of their steric and electrical characteristics, NHCs have become popular ligands [131]. Combining the NHC ligand family with copper became a clear and fruitful field for some. Several methods have been created in the recent decade alone. There are two types of copper-NHC complexes: neutral mono-NHC and cationic bis-NHC derivatives: [Cu(X)(NHC)] (X = halide, acetate, hydroxide, hydride, etc.) and [Cu(NHC)(L)][Y] (L = NHC or PR3; Y = PF6, BF4).Because of their ease of synthesis, neutral-halide-bearing complexes have been widely used in catalysis. Aside from halide-bearing complexes, the following significant related compounds have been reported: The first hydroxide derivative [Cu(OH)(IPr)] was discovered by Nolan and colleagues. (IPr = N,N'-bis(2,6-di-isopropylphenyl)imidazol-2-ylidene) and Sadighi published alkoxides, hydrides, and borate species, allowing for discovering new reactivity. In the case of cationic derivatives, homoleptic and heteroleptic bis-NHC complexes have been reported and successfully employed in catalysis, resulting in significant improvements [132].
2.6.2.1. Alkenes and Allenes Functionalization
2.6.2.1.1. 1,4-Reduction
The 1,4-reduction of enones and enoates is definitely one of copper's most famous reactions. NHC-copper complexes were successfully employed in the conjugate additions of diethyl zinc to enones after Arduengo's pioneering work. Buchwald and Sadighi published the conjugate reduction of α, β-unsaturated carbonyls using a Cu-NHC system in 2003, based on their prior study concentrating on phosphine complexes. The silane sources had no substantial effect in the original investigation of cyclic enones, as yields were nearly identical. THF or toluene can be utilised to conduct this transition efficiently and without losing reactivity. Surprisingly, the reactivity was radically different regarding the type of the α,β-unsaturated ester. The reduction of ethyl trans-β-methylcinnamate was incomplete after 20 hours; thus, an alcohol solvent was added to speed up the reaction. With only 0.3 mol percent catalyst, complete conversion was achieved after only 1 hour in the presence of 4 equivalents of tert-butanol. The researchers looked into a variety of α and β-unsaturated carbonyls. In the presence of [Cu(Cl)(IPr), tri-substituted cyclic enones were also transformed. The phosphine hydride complex needed a high catalyst loading and a long reaction period. There was strong resistance to functional groups like nitro, with no breakdown. Tetra-substituted olefins were successfully used in the process. The creation of a hydride species in situ via -bond metathesis between [Cu(Ot Bu)(IPr)] and the silane was supported by insights into the catalytic cycle. A copper enolate intermediate was generated due to the conjugate reduction (Fig. 21). The copper enolate is protonated in the alcohol process, releasing the alkoxide intermediate and the finished product. Riant and Nahra described a dual catalysis 1,4-reduction/allylation method involving copper and palladium in 2013. [Cu(Cl)(IMes)] was used for the 1,4-reduction process in this example [133]. The allylic alkylation occurred in the presence of a palladium complex, resulting in a diverse set of functional molecules. A hydride intermediate was postulated as part of a mechanism comparable to the conjugate reduction reaction.
2.6.2.2. Conjugated Additions
2.6.2.2.1. Zinc Reagents
Organozinc reagents are frequently used in the field of conjugate addition. Alexakis has made a seminal contribution in this area by developing a phosphoramidite copper-based technology that allows for asymmetric conjugate addition. Woodward described the first effective copper-NHC system capable of 1,4-addition. Exposing cyclohexenone to ZnEt2, a copper source, and SIMes (N,N'-bis[2,4,6-(trimethyl)phenyl] imidazolin-2-ylidene) ligand resulted in rapid synthesis of the conjugate product. This was the initial step toward one of organocopper chemistry's most well-known benchmark reactions. A chiral variant was soon discovered. Poor enantioselectivity ranged from 23% to 50% [134]. According to Alexakis, transmetallation from silver to copper is used in the alternate approach. Enantioselectivity of up to 93 percent was achieved using only 4 mol% copper salt and a silver complex. Mauduit and Clavier published a paper in 2005 describing a new class of chiral alkoxy-imidazolium salts. In relation to temperature, an unexpected phenomenon was seen with Cu(OTf)2 and Cu(eaa)2 (eaa = ethylacetoacetate). Indeed, using Cu(eaa)2 to lower the temperature to -78 °C resulted in a modest loss in enantioselectivity, whereas a considerable decline was observed at ambient temperature. Cu(OTf)2 has the odd result of having the opposite effect.
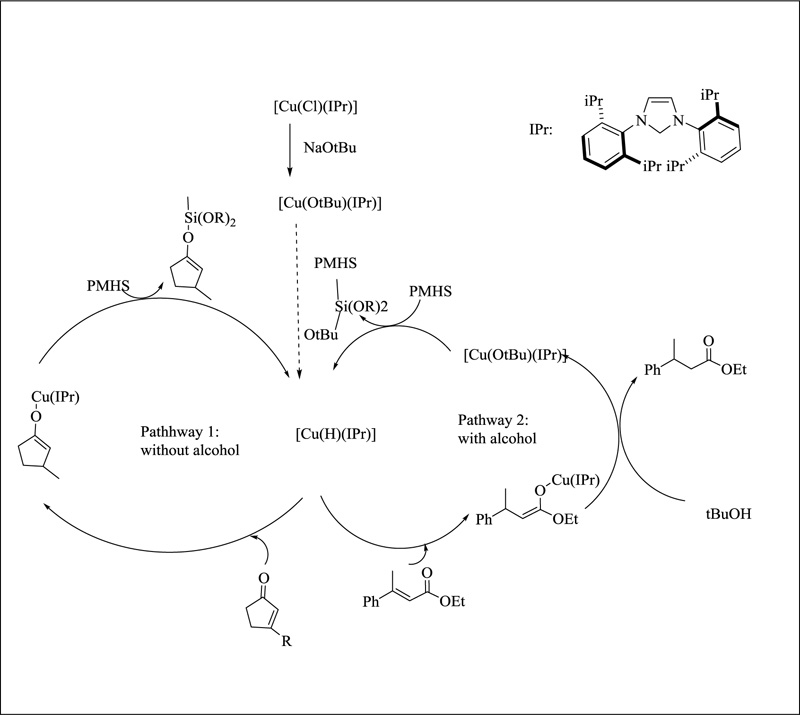
2.6.2.3. Carboxylation of Alkenes
In carboxylation catalysis, copper-NHC complexes have been used. CO2 as a C1 source and chemical feedstock is a hot topic. A number of procedures have been documented that allow carboxylic acids or esters to be formed. In the case of alkenes, however, a tandem reaction has been observed, with in situ productions of alkylborane followed by carboxylation. With potassium tert-butoxide and [Cu(Cl)(IPr), only 35% of the product was seen at 70°C in an ambient CO2 environment. The higher yield was achieved by switching the base to LiOMe, which is less bulky, and practically quantitative yield (97%) was produced with 3 mol percent of catalyst.KOMe was shown to be slightly less effective than NaOMe or LiOMe when the influence of the cation was studied. Because of the intermediate's reduced stability, the less bulky [Cu(Cl)(IMes)] only converted 41% of the time. Surprisingly, a wide range of functional groups, including propargyl, carbonyl, halides, and vinyl bromide, are tolerated. (Fig. 22) Despite these promising results, diphenylethylene remained unresponsive. The hypothesized mechanism involves the production of a boryl copper species followed by the insertion of CO2, comparable to the proposed catalytic cycle for carboxylation of boronic esters [135].
2.6.2.4. Semi-hydrogenation of Alkynes
The semi-hydrogenation of alkynes to produce (Z)-alkenes is a valuable technique. For this reaction, heterogeneous catalysts have been routinely used. The Lindlar catalyst (Pb-poisoned Pd) is the most well-known example. However, this approach has several flaws, including Z/E isomerisation, limited chemoselectivity, and lack of reproducibility. Tsuji reported a CuNHC approach (Fig. 23) for the semi-hydrogenation of non-polar carbon-carbon triple bonds a few years ago [136]. Alkenes were successfully reduced from terminal alkynes. IPr, IPrMe, and IPrCl ligands were used to assess the NHC impact. The NHC-based complexes [137] outperformed the Xanphos ligand in terms of activity, particularly [Cu(Cl)(IPrCl)], which produced styrene (92 percent yield) without forming ethylbenzene.
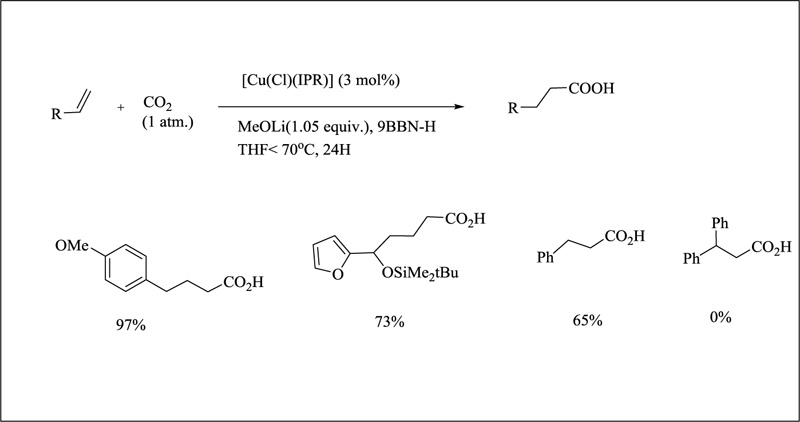
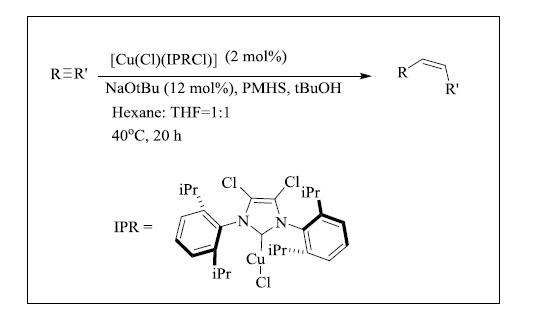
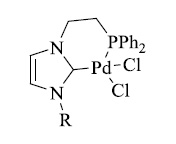
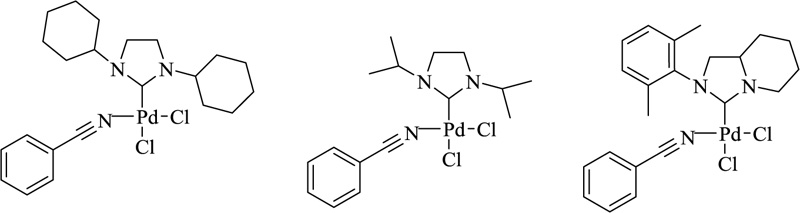
2.6.3. Pd-NHC Complexes
Several Pd-NHC has been reported so far. The diverse coordination and versatile functions of NHC-derived ligands prompted their use in organometallic chemistry and catalysis. Pd-NHC complexes are desirable catalysts in Suzuki and Heck reactions in heterogeneous and homogenous media. Pd-NHC complexes are known to catalyze several C-C coupling reactions. The nature of the Pd ion and the configuration of substituents at the appropriate position is essential. Among various heterocycles, benzimidazole and imidazole-derived NHCs are being explored to a greater extent. Cross-coupling chemistry constitutes one of the most well-defined fields of catalysis research. NHCs are generally considered alternatives to phosphine ligands. Moreover, the effect of substitution at the nitrogen atom of the NHC core, which can be a triazole, tetrazole, imidazole, benzimidazole, or any other nitrogen heterocycle, alters the catalytic behaviour of the Pd-NHC complexes. Pd-NHC complexes have gained much attention because of their electronic and steric implications that affect the polymerization of various olefins like ethylene, norbornene, propylene, etc. The polymerization of cyclic allenes and olefins is one of the most prominent objectives to be achieved in polymer chemistry. There is a difference in the catalytic behavior of double and single-site Pd-NHC complexes in cross-coupling reactions. Using different nitrogen heterocycles like triazole, imidazole, tetrazole, pyridine, benzimidazole, and benzothiazole in the NHC complexes is preferably used in the diverse processes ranging from polymerization to C-C coupling. Apart from cross-coupling reactions, Pd-NHC complexes are suitable catalysts in oligo/polymerization and act as anti-cancer agents in certain cell lines. The stereochemical aspects governing double and single-site Pd-NHCs provide a critical review of these complexes from a synthetic point of view [138].
2.6.3.1. Single Site Pd-NHC complexes
Single-site Pd NHCs have emerged as potential precursors in the design of catalysts for several organic reactions. Hexa or tetra-coordinated derivatives of Pd with NHCs provide a better route to enhancing the applications of Pd-NHC complexes and extension of supramolecular chemistry in biomedical fields. Several researchers recently reported synthesizing and characterizing various single-site tetra-coordinated Pd derivatives (Fig. 24), exhibiting C-C coupling via metal ions. The kinetic and thermodynamic properties of the process are being analyzed via various spectroscopic methods, including UV, nuclear magnetic resonance (NMR), Fourier Transform infrared spectroscopy (FTIR), etc. The preliminary results indicated the use of monometallic NHC complexes in C-C coupling and synthesis of new catalysts. Many single-site NHC complexes have been synthesized via simpler methods, and catalyst libraries have already been designed [139].
2.6.3.2. Imidazole-based NHC complexes
Imidazole-based ligands coordinate with high and low oxidation state transition metals such as palladium, nickel, chromium, molybdenum, rhenium, etc. Imidazole-based Pd-NHC complexes (Fig. 25) have gained quite some interest in recent times concerning C-C coupling. Although the ligands are not significant in inducing the chirality, they amplify the related characteristics provided the reaction is chiral. A very important class is tetra ether-NHCs since these attempts to tetra-hydroxy and dialdehyde upon reaction with dilute acids. Tetra ether NHC complexes also show good catalytic action in the Heck reaction. Dithicyanato, dihalo, and tri-fluoroacetato substituted NHC complexes possessing ethylene/methylene bridged NHC ligand system catalyze various reactions like Suzuki reaction, Heck Reaction, etc. Stereochemistry, however, is essential in the efficiency of catalysis. The catalytic behavior of imidazole-based NHCs depends not only on the nature of the substituents but also on the steric effects of those substituents. Many imidazole-based Pd-NHC allyl complexes having different ancillary ligands acting as catalysts have also been reported [140].
2.6.3.3. Triazole-based NHC Complexes
Certain single-site triazole-based Pd-NHC complexes like 1,2,4 trisubstituted triazole derivatives serve as NHC precursors. Recently, 1,3,4-tri and 1,4-disubstituted triazoles have gained interest since many of those complexes serve relatively well catalysts in various organic synthetic reactions. Tetrazole-based NHC complexes are comparatively fewer, but their catalytic activity is highly appreciated [141, 142].
CONCLUSION
The extent to which heterocycles are being invented and tested for their applications determines the vitality and strength of this domain of organic chemistry. The challenges in synthesizing new heterocycles and evaluating their properties are responsible for thriving research in this area. N-heterocycles are of utmost importance in this regard since a majority of heterocycles possessing varied applications possess nitrogen as a heteroatom. The nitrogen heterocyclic framework offers an elevated scope for structural diversity that has proved useful in improving their physiochemical and pharmacokinetic properties. The overwhelming advantage of nitrogen heterocycles includes high availability, easy preparation, and good biocompatibility. This review article aims to provide valuable insights into designing and developing effective and sustainable nitrogen-based compounds in various fields.
LIST OF ABBREVIATIONS
MCF | = Michigan Cancer Foundation |
DMF | = Dimethylformamide |
DHFR | = Dihydrofolate reductase |
SAR | = Structure-activity relationship |
DFT | = Density functional theory |
LUMO | = Lowest unoccupied molecular orbital |
HOMO | = Highest occupied molecular orbital |
PET | = Photoinduced electron transfer |
NIR | = Near infra-red |
NHC | = Nitrogen heterocyclic carbene |
CONSENT FOR PUBLICATION
Not applicable.
FUNDING
None.
CONFLICT OF INTEREST
Praveen Kumar Sharma is the Editorial Advisory Board of The Open Medicinal Chemistry Journal.
ACKNOWLEDGMENTS
We would like to express our gratitude to Lovely professional University, Phagwara, Punjab, India, for providing us with the necessary books and e-resources.