All published articles of this journal are available on ScienceDirect.
The Effects of Foliar Spray of Chitosan Nanoparticles on Tomato Resistance Against of Cuscuta Campestris Yunck
Abstract
Background:
Crop yield is limited by several factors. One of these factors is biotic stress. Among biotic stresses, parasitic plants have the greatest role in reducing plant growth and productivity. On the other hand, the multiple uses of pesticides and herbicides have a negative impact on the environment and agricultural products. As an eco-friendly compound, chitosan stimulates the defense system, increases plant resistance to biotic and abiotics stresses, and increases crop growth and productivity.
Methods:
In this study, the tomato plant was infected with Cuscuta campestris Yunck and sprayed with chitosan nanoparticles with a molecular weight of 50 kDa for 4 weeks and once a week. Four weeks after the infection, plants biomass, protein concentrations, antioxidant enzyme activity (SOD, CAT, APX, POD), and phenolic metabolism (PAL, PPO, total phenolic components, lignin) were measured.
Results:
In this study, chitosan application increased biomass in Dodder-infected and non-infected plants. The concentration of soluble proteins also increased. In Dodder-infected plants, the activity of antioxidant enzymes increased and the application of chitosan further increased the activity of these enzymes. Phenol metabolism as a defense mechanism in Dodder-infected plants increased compared to the control and chitosan application intensified phenol metabolism. SDS-PAGE analysis investigated that strong bands are formed in the range of 200 and 60 kDa in chitosan treatments and chitosan-treated infected plants.
Conclusion:
These bands were also observed in Dodder-infected plants that were not treated with chitosan.
1. INTRODUCTION
Parasitic plants are the most problematic pests of crops [1]. They are extremely morphologically diverse and range from diminutive herbaceous plants to large trees, as well as highly reduced parasites that grow embedded in their host and lack leaves and roots. Parasitic plants can be divided according to whether they are photosynthetically active or lack photosynthetic activity, and whether they are completely attached to a host for carbon, whether they are optional or obligate parasites, and whether they are attached to the roots or stem of the host. In natural ecosystems, parasitic plants form a component of the plant community and contribute to the overall community balance of parasitism. Conversely, when parasitic plants are established in low biodiverse agroecosystems, their persistence causes enormous yield losses and renders agricultural land inoperable. It has been determined that many features, from seed germination to haustorium formation, from feeding patterns to host selection, are based on the relationships between the host and the parasite [2].
Many problems such as diseases, weeds and pests are encountered in greenhouse, and field tomato cultivation. Dodder (Cuscuta campestris) is one of the most important pests of tomato causing severe losses in yield. If no action is taken to control the Dodder (Cuscuta campestris), economic loss occurs 100% in tomato yield and quality [3]. This parasite has a wide range of host species [4-6]. It mainly parasitizes alfalfa but also attacks some horticultural crops, legumes, and broadleaved weeds, though it is seldom found on woody plants, grasses, or cereals [7].
Chitosan to cover products, such as tangerines, strawberries, and cherry, is used. In addition to being edible, this substance is able to show antibacterial activity. Low molecular weight chitosan effect has significant anti-fungal activities and is more effective in maintaining the quality of citrus fruits [8]. By using nanoclay in biopolymer, good barriers can be created against the penetration of gases and water. Many studies on the effect of nanoclay on reducing the penetration of oxygen and water vapor have been reported [9-11]. The use of polymers impregnated with clay nanoparticles in the packaging industry increases the time it takes for air to reach agricultural products and delays its spoilage. In this study, we try to investigate how chitosan foliar spray with a molecular weight of 50 kDa stimulates the antioxidant defense system of the tomato plant to increase the parasitic resistance of the Cuscuta campestris [12, 13].
2. MATERIALS AND METHODS
2.1. Plants Material and Treatments
Tomato (Solanum lycopersicum L.) seeds and Dodder (Cuscuta campestris Yunck) were sterilized by 10% sodium hypochlorite for 5 minutes and planted together in a pot on a perlite bed in growth chamber with 16 h light (350 mol photons m˗2s˗1) at 26°C and 8 h dark at 20°C, both at 50% humidity. After germination of seeds and successful infection with the Dodder, the plants were irrigated 100% by Hoagland for 4 weeks. During this time, the plants were sprayed once a week with 10 ml of 50 kilodalton (kDa) (50 mg ml˗1) chitosan (Sigma-Aldrich CAS-No 9012-76-4) and distilled water was used as a control.
2.2. Determination of Total Protein Concentration
Protein content was determined by the method of Bradford utilizing bovine serum albumin as the standard [14].
2.3. Enzymes Assay
Plants material was homogenized in 3 ml of 0.05 M sodium phosphate buffer (pH 7.8), including 1 mM EDTA and 2% (w/v) PVP. The homogenate was centrifuged at 10,000 × g for 10 min at 4 ̊ C. Supernatant was used for enzyme activity and protein content assays. All steps in the preparation of the enzyme extract were carried out at 4°C [15].
SOD activity assay was based on the method described by Giannopotitis and Ries [16]. One unit of enzyme activity was defined as the amount of enzyme required to result in a 50% inhibition of the rate of nitro blue tetrazolium reduction measured at 560 nm.
Ascorbate peroxidase (APX) activity was determined according to Nakano and Asada [17], the reaction mixture in a total volume of 2 ml consisted of 25 mM (pH 7.0) sodium phosphate buffer, 0.1 mM EDTA, 0.25 mM ascorbate, 1.0 mM H2O2 and 100µl enzymes extract [18]. H2O2-dependent oxidation of ascorbate was followed by a decrease in the absorbance at 290 nm.
Catalase (CAT) activity was done according to Cakmak and Marschner. The reaction mixture in a total volume of 2 ml contained 25 mM sodium phosphate buffer (pH= 7.0), 10 mM H2O2. The reaction was initiated by the addition of 100 µl of enzyme extract and activity was determined by measuring the initial rate of disappearance of H2O2 at 240 nm.
Peroxidase (POD) activity was measured using modification of the procedure of McAdam et al. [19]. Guaiacol was used as the substrate. POD activity was measured in a reaction mixture (3 ml) that contained 0.1 ml enzyme extract, 12 mM H2O2, and 7.2 mM guaiacol in 50 mM phosphate buffer, pH 5.8. The kinetics of the reaction were followed at 470 nm.
PAL activity was assayed by an adaptation of the methods of Zucker [20] and McCallum and Walker [21]. The assay mixture consisted of 0.06 M Na borate buffer, pH 8.8, and crude enzyme. The reaction was started by the addition of 11 mM L-phenylalanine. Tubes were incubated at 30°C for 60 min and the reaction was stopped by the addition of 35% (w/v) trifluoroacetic acid (TFA). Tubes were then centrifuged for 5 min at 5000rpm to pellet the denatured protein. Phenylalanine ammonia lyase (PAL) was enzymatic, and a sample extract was boiled and assayed.
PPO activity was assayed as described by Nicoli et al. with some modifications [22]. Optimum activity was reached using SDS at 2% (data not shown). The assay mixture consisted of 30 mM caffeic acid in 100 mM buffer (Na2HPO4/KH2PO4), pH= 7.0, through which air was bubbled for 5 min. Catalase (420 units) from bovine liver (Fluka) was added in 0.1 ml H2O to prevent peroxidation of the substrate. The assay was initiated by the addition of enzyme extract. Polyphenol oxidase (PPO) activity was measured by the change in A370 of the assay mixture (30°C) based on the measurement of the disappearance of caffeic acid by enzymatic oxidation. To determine whether the reaction was enzymatic, the sample extract was boiled and assayed.
Total phenol content was estimated by the method suggested by using Folin–Ciocalteu reagent (1 N) and the absorbance was measured at 650 nm against each blank [23]. The content of phenol was calculated from the standard curve obtained from different concentration of catechol and expressed as mg g ˗1 FW.
2.4. Lignin Quantification
Lignin was quantified by the thioglycolic acid (TGA) method described by Lange et al. [24] and adapted by Torre et al. [25]. Tubes containing about 100 mg of plant material were treated with 1 ml of methanol, shaken for 1 h, and centrifuged at 10,000 g for 10 min. The supernatant was removed and the pellet was treated successively with 1.5 ml of the following solvents: methanol, 1 M NaCl, 1% (w/v) Sodium dodecyl sulfate (SDS), deionized water and chloroform: methanol (1:1). Finally, the pellet was resuspended in 1.5 ml of tert-butyl methyl ether and lyophilized. 10 milligrams of the resulting powder was resuspended in 1 ml of 2 M HCl and 0.2 ml of thioglycolic acid and incubated at 95 ̊ C for 4 h. The solution was centrifuged at 10,000 g for 10 min and the resulting pellet was washed three times with deionized water, resuspended in 1.5 ml of 0.5 M NaOH and shaken for 12 h. The samples were then centrifuged at 10,000 g for 20 min, and the supernatant was mixed with 0.3 ml of 12 M HCl and incubated for 4 h at 4 ̊ C. After centrifugation for 40 min, the resulting pellets were dried using a speed vacuum concentrator and resuspended in 1 ml of 0.5 M NaOH. The A280 was measured and the concentration was calculated with a previously determined lignin standard made with pure lignin from Sigma-Aldrich.
2.5. SDS-PAGE Technique
The total cell proteins were subjected to Sodium dodecyl sulfate polyacrylamide gel electrophoresis (SDS-PAGE) in gel slabs 1 mm thick (3.5 cm, 4% stacking and 15.5 cm, 12% resolving gels) as mentioned by Laemmli [26]. Electrophoresis was carried out with a discontinuous buffer system in a UVP vertical electrophoresis chamber (Cambridge, UK). The gel was run at 30 mA until the bromophenol blue marker had reached the bottom of the gel. The molecular weight of proteins was calculated on the basis of comparison with the following standards (Thermo Scientific Page Ruler Protein Ladder SDS-PAGE Standards, Fermentas, molecular weight range 10-200 kilodalton). After electrophoresis, the gels were stained for 6 h in 0.01% (w/v) Coomassie Brilliant Blue R-250. Finally, the gels were destained in a methanol acetic acid-water (3:1:6) mixture until protein bands became clearly visible.
3. RESULTS AND DISCUSSION
3.1. Biomass
In this study, the biomass of the Dodder-infected tomato plant was significantly reduced compared to the not-infected plant. Chitosan foliar spray increased biomass in both infected and non-infected plants, indicating an improvement in plant conditions (Fig. 1). Chitosan has a positive effect on plant growth and productivity and increases plant yield by improving plant condition, especially in stressful conditions [27]. Not-infected with Dodder (Control), data represent the mean ± SD with n =4 for each treatment, * letters show significantly different values after one-way ANOVA followed by Duncan’s multiple range test (p ˂ 0.05).
3.2. Proteins Concentrations
In this study, the application of chitosan caused protein concentrations in not-infected and Dodder-infected plants increase which indicates an increase in the expression of defense enzymes and pathogenesis-related (PR) genes/proteins (Fig. 2). Studies have shown that the use of chitosan increases the concentration of proteins in plants that are related to the increased expression of defense system enzymes and genes or proteins related to pathogenicity.
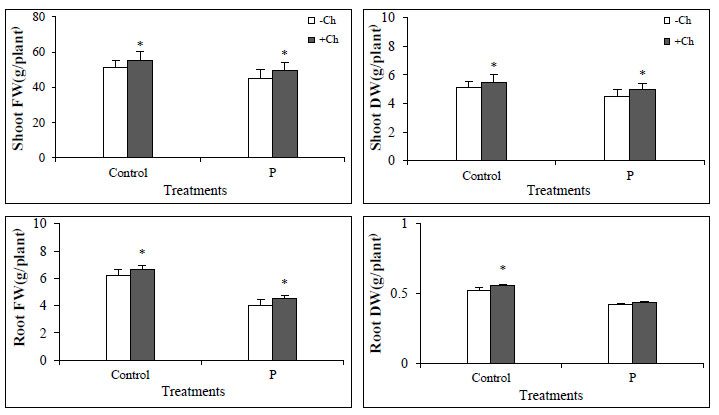
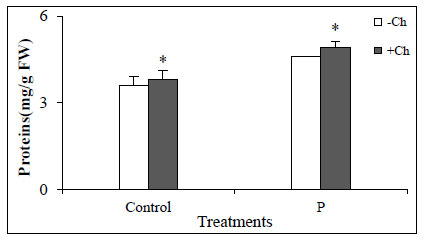
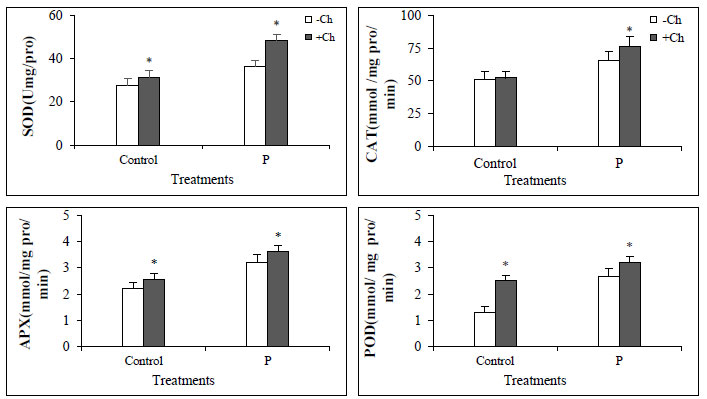
Generally, the activity of antioxidant enzymes increased in Dodder-infected plants compared to control, and in plants that were both infected and treated with chitosan, the enzyme activity was much higher (Fig. 3). The activity of antioxidant enzymes increases under stressful conditions and reduces the oxidative damage by decreasing active oxygen species and increasing plant resistance. Chitosan, as an elicitor, stimulates the plant's defense system and thus increases the plant's resistance to the pathogen [28, 29].
3.3. Phenolic Metabolism
Phenylalanine ammonia lyase (PAL) activity was significantly increased in infected plants compared to control. This increase in enzyme activity was also observed in plants treated with chitosan. However, in plants that were both infected and treated with chitosan, the enzyme activity was much higher. In the case of PPO, the activity increased in infected plants and the application of chitosan made the enzyme more active in both infected and non-infected plants. The same pattern was observed for phenolic and lignin compounds (Fig. 4). Biostimulants such as chitosan cause the production of phenolic compounds including lignin. Phenolic compounds can act as antioxidants by removing ROS from plant cells [30-32].
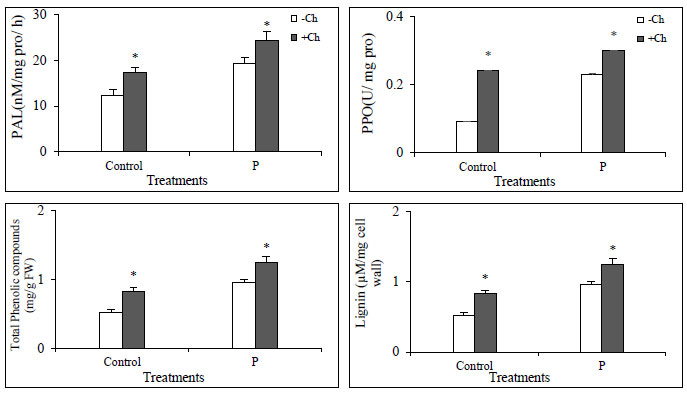
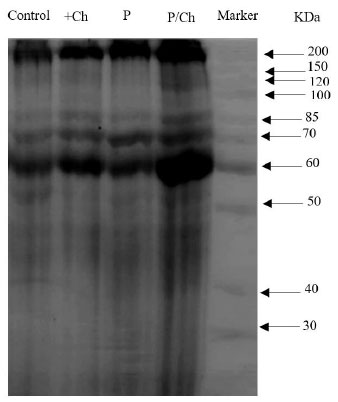
3.4. SDS PAGE Analysis
The findings of SDS-PAGE showed that the levels of 2 protein bands of molecular masses 200 kDa and 60 kDa gradually increased in Dodder-infected plants treated with chitosan. We suggested that these proteins may be related to pathogenesis. In brief, the findings of SDS-PAGE revealed that several proteins are involved in plant defense response. However, in the treatment of chitosan alone and also in infected plants that have not been treated with chitosan, the intensity of these bands is less (Fig. 5).
4. CONCLUSION
Recently, studies have focused on the use of compounds that do less harm to the environment while increasing the yield of agricultural products. One of these compounds is chitosan, which has received much attention from scientists. In this study, chitosan foliar spray with a molecular weight of 50 kDa and a concentration of 50 mg ml˗1 had a positive effect on biomass and plant defense system, and through this, Dodder-infected plants treated with chitosan showed greater resistance.
LIST OF ABBREVIATIONS
KDa | = Kilodalton |
CAT | = Catalase |
POD | = Peroxidase |
APX | = Ascorbate Peroxidase |
PAL | = Phenylalanine Ammonia Lyase |
RPM | = Revolutions Per Minute |
PPO | = Polyphenol Oxidase |
SDS | = Sodium Dodecyl Sulfate |
SDS-PAGE | = Sodium Dodecyl Sulfate Polyacrylamide Gel Electrophoresis |
ETHICS APPROVAL AND CONSENT TO PARTICIPATE
Not applicable.
HUMAN AND ANIMAL RIGHTS
Not applicable.
CONSENT FOR PUBLICATION
Not applicable.
AVAILABILITY OF DATA AND MATERIALS
The data that support the findings of this study are available within the article.
FUNDING
None.
CONFLICT OF INTEREST
The authors declare no conflict of interest, financial or otherwise.
ACKNOWLEDGEMENTS
The authors are grateful to the research council of the Payamnoor University for this study.