All published articles of this journal are available on ScienceDirect.
A Detailed Analysis of the Carotenoids and their Derivatives, including their Multiple Health Advantages
Abstract
Background:
The majority of foods, vegetation, plankton, and photosynthetic bacteria all include carotenoids, which are pigments that naturally exist. Since humans are unable to produce carotenoids, they must be consumed through food or supplements. There are many roles for carotenoids in ensuring human health. They generally act as antioxidants, but different carotenoids may also have other functions. For instance, β-carotene functions as pro-vitamin A, while lutein and zeaxanthin make up the macular pigmentation in the eyes.
Objective:
The primary goal of this review article is to emphasise the numerous health advantages of the various carotenoids and their derivatives, including neuro, cardio, bone, immune, and cancer protection.
Methods:
The information for this review article was acquired by using Google Scholar and PubMed as search engines, as well as a number of publishers, including Springer Nature, Bentham Science, Taylor & Francis, and Elsevier.
Results:
Carotenoids are biologically active antioxidants, and their anti-inflammatory and antioxidant properties may help to lessen cardiovascular events such as adiposity, insulin sensitivity, hyperlipidemia, and inflammatory markers. Evidence shows they enhance eyesight, cognitive ability, and cardiovascular health. They could also reduce the risk of a number of malignancies. Carotenoids' diverse properties and therapeutic capacity to prevent neurodegeneration in conditions including Alzheimer's, Parkinson's, stroke, and others are reviewed.
Conclusion:
Despite the data supporting the health advantages of carotenoids, extensive population-based supplement research on several carotenoids has yielded conflicting results. Further study, especially clinical studies, is required to demonstrate and validate the medical benefits of the various carotenoids.
1. INTRODUCTION
Carotenoids are naturally occurring, fat-soluble, strongly unsaturated oranges, a yellow, red pigment found in fungi, bacteria, plants, and algae. The amount of carotenoids present is typically correlated with the intensity of colour. Vegetables and fruits naturally contain large amounts of carotenoids. Additionally, some bacteria and photosynthesis-producing algae are reliable sources of these chemicals. The isoprenoids, including the phytochemical carotenoids, have a fundamental structure comprising eight isoprene units with a C40 backbone [1]. Carotenoids have a variety of physiological functions, including antioxidant activity, and their presence in the body is crucial for good health. As a result, eating foods high in carotenoids can help prevent and treat several illnesses, including cancer, age-related macular degeneration, cardiovascular disease, and neurodegenerative diseases [2-6]. They have beneficial effects on eye health, cognitive function, and cardiovascular health and may help reduce the risk of some types of cancer. This is especially an issue for lutein, which has demonstrated health benefits in reducing age-related macular eye disease progression. Carotenoids are a naturally occurring lipophilic secondary metabolite with significant anti-NDD therapeutic potential. The variety of carotenoids and their healing potential for reducing or preventing immunological, cardiovascular, and neurodegenerative disease. In this specific article, we have detailed the numerous health advantages of the different carotenoids [7].
2. CAROTENOIDS
Carotenoids are colourful, liposoluble pigments. They are present in many foods, such as vegetables, fish, and fruits, as well as in plants, fungi, bacteria, and algae. More than 600 carotenoids have natural structural variations, which are grouped into carotenes, xanthophylls, and lycopene [8, 9]. Only 40 carotenoids make up the average human diet, while only 20 have been found in human tissues and blood. Because they have many chloroplasts, where most carotenoids are found, darkish green vegetables like coriander, broccoli, spinach, and kale have high levels of carotenoids [10]. The body and food contain the carotenoids β-carotene, α-carotene, lycopene, lutein, and cryptoxanthin. Tetraterpenoids, or carotenoids, are C40 hydrocarbons with isoprenoids as their basic constituents. The carotenoid varieties are all formed from the skeleton and are what give fruits, foliage, and flowers their characteristic yellow, orange, or red colour. The C40 carbon skeleton of carotenoids is created by combining two C20 geranylgeranyl diphosphate molecules [11]. Most carotenoids include diverse cyclic or acyclic end groups and core alternating single and double bonds in a carbon chain [12].
Carotene is the major carotenoid found in bovine milk [13]. However, astaxanthin and canthaxanthin are the two main carotenoids found in salmonid fish and crustaceans [14, 15]. In addition, fucoxanthin, a significant carotenoid, is present in several brown edible seaweeds [16, 17]. Several organs in the human body have different distributions of carotenoids. It's interesting to note that in the frontal and occipital lobes of the human brain, xanthophylls make up 66-77% of all the carotenoids [18], whereas most tissues and plasma contain less than 40% of the total carotenoids [19-21].
2.1. Chemistry of Carotenoid
The main carotenoids, Lycopene, alpha-carotene, ASTA (astaxanthin), lutein, and zeaxanthin, are the primary carotenoids. By connecting two C20 geranylgeranyl diphosphate molecules, carotenoids are created. A lengthy conjugated chain of double bonds, similar to bilateral symmetry, and a polyisoprene structure are all present in all carotenes. Pro-vitamin A (like α-carotene, β-carotene, and β-cryptoxanthin) and non-provitamin A molecules are two categories of carotenoids. In comparison, carotenoids can be divided into two groups based on the functional groups they contain: (a) xanthophylls (such as lutein and zeaxanthin), which contain oxygen as a functional group, and (b) carotenes (such as α-carotene, β-carotene, and lycopene), which always contain a parent hydrocarbon chain and no other functional groups. By `employing carotenoid cleavage dioxygenases, other molecules like apocarotenoids (e.g., retinoids, vitamin A, α-ionone, and β-ionone aromatic volatile chemicals) are also produced from carotenoids [22]. Carotenoids often function in hydrophobic regions of the cell and are hydrophobic molecules with very poor water solubility. Polar functional groups can alter carotenoids' polarity added to polyene chains, which may have an impact on where they are located in biological membranes and how they interact with other molecules [23]. Carbon, hydrogen, and oxygen atoms make up the hydroxyl, epoxide, and keto groups in xanthophylls [24, 25]. Enzymatic processes involving hydroxylases, epoxides, or synthases lead to the formation of xanthophylls. Carotenoids are xanthophylls with a hydroxyl group (such as lutein, zeaxanthin, and β-cryptoxanthin), whereas epoxy carotenoids are xanthophylls using an epoxide group (such as violaxanthin, neoxanthin, antheraxanthin, 6-epoxide, α-carotene β-carotene-5 [26]. (Table 1, Fig. 1).
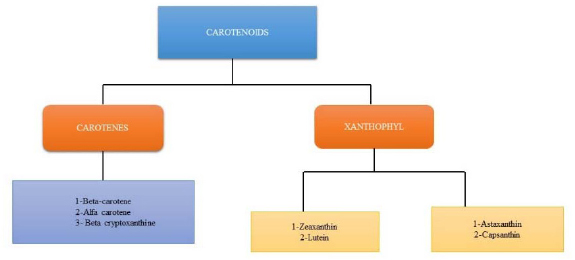
2.2. Classification of Carotenoids
S.No. | Carotenoids | Structure | Food Sources | Pigment | References |
---|---|---|---|---|---|
1 | Astaxanthin | ![]() |
• Algae• Salmonid• Fish | Reddish orange pigmentation | [27] |
2 | Zeaxanthin | ![]() |
• Cucumber• Diary product• Honeydew | Yellow colour Pigmentation | [28, 29] |
3 | Fucoxanthin | ![]() |
• Bown seaweeds | Orange colour pigmentation | [30] |
4 | Lutein | ![]() |
• Apple• Basil• Dairy product• Pumpkin | Xanthophyll pigmentation | [31] |
5 | β Carotene | ![]() |
• Dairy product• Tomato• Spinach• Banana | Red- orange pigmentation | [32] |
6 | Alfa carotene | ![]() |
• Carrot• Butternut• Banana | Orange colour pigmentation | [33] |
7 | β cryptoxanthin | ![]() |
• Papaya• Peach• Tangerine | Orange colour Pigmentation | [34] |
2.3. Biosynthesis of Carotenoids
The mechanism (1-4') of head-to-tail condensation of three isopentenyl diphosphates (IPP) and one dimethylallyl diphosphate (DMAPP) yields the isoprenoid molecule known as geranylgeranyl diphosphate, which is the first step in the carotenoid synthesis process (GGPP). An olefinic double bond of IPP attacks the carbocation created by the generation of the allylic diphosphate group. The enzyme GGPP synthase (GGPPS), which possesses the cyclic condensation mechanism of ionisation-condensation-elimination, catalyses this reaction. A variety of substances, including carotenoids, tocopherols, chlorophyll, plastoquinone side chains, and phytohormones, are precursors to GGPP [35]. Phytoene is a dimerised substance that is created by the head-to-head coupling of two GGPP, which is catalysed through phytoene synthase (PSY) [36, 37]. A chain containing three conjugated double bonds identifies a molecule as a phytoene. Two conjugated double bonds are added to the lengthy chain by the enzyme phytoene desaturase (PDS), creating the phytofluene molecule. The desaturation reaction then results in the formation of two more conjugated double bonds and carotene. Carotene isomerase (CRTISO) or β-carotene isomerase converts the poly-cis-carotenoid molecule produced. Trans-carotenoid is produced during this biosynthetic pathway. (ZISO). The enzyme β-carotene desaturase (ZDS), which adds two conjugate double bonds to the chain, biosynthesises the neurosporene molecule. This desaturase enzyme continues the catalytic process to create the lycopene molecule by adding two conjugate double bonds. Porter and Lincoln reported the cyclisation of lycopene, positing that cyclisation might occur on one or both sides of the carotenoids to produce several compounds. Lycopene cyclisation produces two unique rings: β- or ε-ionone rings. Only a few alterations often occur along the carotenoid's lengthy chain, with the main modification typically occurring. Lycopene cyclase (LCY), an enzyme that is present in cyanobacteria and plants, cycles lycopene. The LCY subtype, namely the β-ionone (LCYB) rings, ε-ionone (LCYE) rings, or bifunctional β-ionone (LCYB/E) rings, determines the kind of carotenoid. An unsubstituted -ionone ring is thought to provide a source of vitamin A, a chemical crucial to human health. Lycopene changes into -carotene when LCYB is present. In this way, LCYE causes lycopene to change into β-carotene. A six-member ring is present at the end of the chain in both compounds. The molecule ϒ-carotene can be converted by LCYB into ϒ-carotene [35]. While apocarotenoids lack C40 atoms, they share a structure with carotenoids. The two most well-known apocarotenoids are apo-12'-capsorubin and bixin. Apocarotenes are thought to be the result of the oxidative cleavage of carotenoids [38, 39] (Fig. 2).
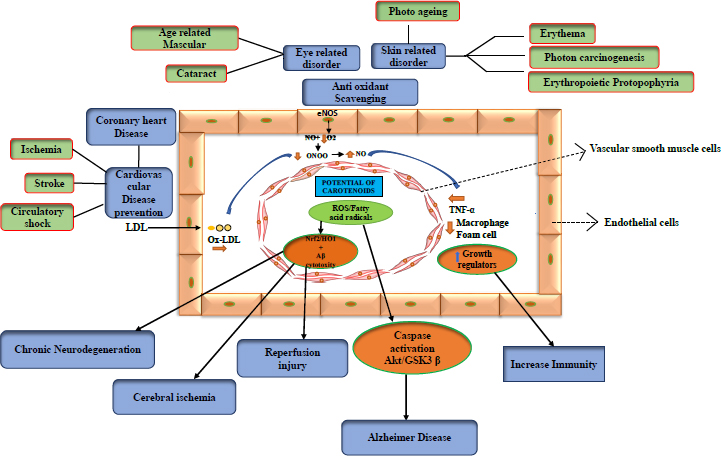
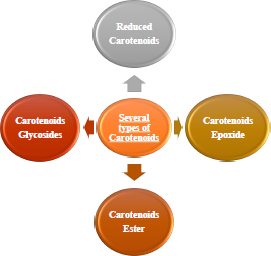
2.4. Carotenoids Derivative
A good substitute for including this molecule with several therapeutic effects in an aqueous medium is synthesising carotenoid derivatives with hydrophilic qualities [40, 41]. Since most carotenoids are a source of vitamin A, contain antioxidant molecules such as anti-carcinogenic capabilities, and have the capacity to fend off CVD, they have a significant nutritional benefit (Fig. 3) [42, 43]. It is essential to synthesise hydrophilic derivatives of carotenoids since they can be used as medicinal compounds to increase the biological activity of carotenoids. The improved water solubility of synthetic carotenoid derivatives is another justification for their production. The widespread usage of carotenoids as natural colours in the food business.
3. POTENTIAL THERAPEUTIC BENEFITS
Carotenoids, which are food-based, are thought to improve health by reducing the risk of illness. One of the most vital nutrients found in green leafy vegetables (e.g., spinach, peas, lettuce, and broccoli), as well as other foods like eggs, is lutein, a carotenoid molecule. Lutein, a nutritional component, imparts benefits against various health conditions, including neurological illnesses, skin irritation, eye disease, etc. Nutrition plays a crucial role in sustaining human health. The benefits of lutein are that it acts against neurological problems, ocular ailments, heart issues, microbiological infections, skin irritation, and bone loss [44].
3.1. Neuroprotective
Alzheimer's, Huntington's, Parkinson's, and amyotrophic lateral sclerosis (ALS) are examples of neurodegenerative diseases. However, carotenoids, including astaxanthin, β-carotene, and lycopene, are engaged in Ca2+ ion transportation in the brain, and with sufficient intake of carotenoids, malfunction due to faulty signalling can be avoided. Several disorders are caused by Ca2+ failure to communicate with molecules. The capacity to pass the blood-brain barrier, stable cell mitochondria, and antioxidant carotenoid-astaxanthin properties may be able to lower the risk of nervous system illnesses (neurodegenerative diseases). In addition to its anti-apoptotic effects, astaxanthin can also minimise cerebral infarction in brain tissue, lower ischemia by inducing apoptosis, reduce glutamate release, and lessen the effects and damage caused by free radicals. [45, 46]. In Alzheimer's disease, ROS increases Akt/GSK-3 signalling and caspase activation. Carotenoids reduce caspase activation and assist in restoring normal signalling. Chronic neurodegeneration is caused by ROS-lowering Nrf2/HO-1 or HO-1, including cytotoxicity, which can cause cerebral ischemia or reperfusion injury [47].
3.1.1. Parkinson Disease
The loss of dopaminergic neurons in the brain's Substantia nigra pars compacta (SNpc), which makes Parkinson's disease (PD) the second-most common neurogenerative disease (NDD) [48], distinguishes it from other diseases of the same type. Excessive ROS accumulation brought on by inflammation or mitochondrial malfunction continues to be a significant component in the decline of dopaminergic neurons. The brain's main sites of ROS formation were glial cells and the mitochondria of neurons [49, 50]. ROS-induced Parkinson's disease (PD) progression is influenced by several factors, including neuroinflammation, ageing, mitochondrial damage, dopamine degeneration, GSH utilisation, extreme exposure to lead, and elevated calcium and iron levels [51, 52]. Dopaminergic neurons were lost in the process of excreting excess neuromelanin because pigmented neurons were more accessible to injury. Additionally, the formation of ROS in PD patients was increased by exposure to environmental variables like neurotoxins and pesticides [53]. PINK1 mutation-related mitochondrial complex A mitochondrial DNA deficit contributes to mitochondrial dysfunction at the time of the start of Parkinson's disease (PD). Dopamine receptors come in five varieties: D1, D2, D3, D4, and D5. Every receptor serves a different purpose. When coupled to the D1 receptor, dopamine aids in controlling neuronal growth. While D2, D3, and D4 receptors aid in signalling for human dopamine neurons' ability to survive and neural growth, D1 and D5 receptors work together to regulate memory, learning, and motor activity [54]. In the hippocampus and substantia nigra, the thickness of GABA receptors and dopamine receptors (D1, D2) typically decreases throughout PD [55].
Carotenoids target dopamine receptors to provide neuroprotection. To study the therapeutic effects of lycopene on GABA neurons and dopaminergic receptors (D1 and D2) in the substantia nigra and hippocampus of Parkinson's disease mouse models. In the substantia nigra and hippocampus of mice, lycopene therapy greatly enhanced the levels of GABA and dopamine receptors. Over time, it was discovered that the number of the investigated receptors increased considerably. Therefore, by raising the density of receptors, lycopene lessens PD-related brain damage. Lycopene is therefore suggested as a useful remedy for reducing the degenerative symptoms of PD, as reported by Zabihollah et al. (2015) [56].
The study reported by Ji Hyun Kim et al. (2016) measured the serum levels of retinol, α- and γ-tocopherols, α- and β-carotenes, lutein, lycopene, zeaxanthin, and β-cryptoxanthin were measured and compared them between 104 patients with idiopathic PD and 52 healthy controls matched for age and gender. Compared to controls, PD patients had lower levels of α- and β-carotenes and lycopene. α-carotene, β-carotene, and lycopene levels were significantly reduced in advanced PD patients relative to early PD patients and were negatively scored in PD patients. No significant differences were found between PD patients and controls serum levels of retinol, α- and γ-tocopherols, and other carotenoids. No significant correlations were found between these vitamin levels and clinical variables in PD patients [57].
3.1.2. Alzheimer's Disease
Alzheimer's disease (AD), the most prevalent neurodegenerative disease, is defined by changes in behaviour that impair daily activities and a reduction in motor, cognitive, and sensory skills, including subsequent memory loss [58]. Multiple neurotransmitters are lost; synaptic impulse failure, decreased neuronal metabolism, extracellular amyloid (A) buildup, and intracellular neurofibrillary tangle (NFT) aggregation are all symptoms of AD [59, 60]. Patients in the latter stages of their illness are unable to communicate or respond to their surroundings and must rely on others to carry out their daily tasks. Because the endoplasmic reticulum's capacity to store Ca2+ ions has been exhausted, aggregates of A produce Ca2+ overloading in the cytosol (ER). Reduced expression of glutathione GSH) and a buildup of intracellular reactive oxygen species are caused by higher cytosolic Ca2+ concentrations (ROS) [61]. As increased ROS induces aggregation and the production of A plaques, ROS-induced oxidative stress plays a significant role in the pathophysiology of AD [62].
Carotenoids have been shown to have certain neuroprotective qualities, including reducing the production and accumulation of amyloid plaques as well as having anti-neurotransmission and anti-inflammatory effects [63, 64]. The targeting of these particular nutrients is a function of carotenoids' antioxidant activity, which they achieve by scavenging harmful oxygen radicals [65]. Applying carotenoids may result in decreased amounts of β-amyloid, lowering the risk of AD [66].
Studies found that carotenoids have neuroprotective effects on SH-SY5Y cells by reducing amyloid secretion and shielding the cells from copper and H202-induced oxidative damage [67]. The reduction in β-amyloid-induced mitochondrial damage was also observed in male Wister rats with cognitive impairment after receiving carotenoids, as reported by (Prakash A et al. 2014) [68]. Several studies have suggested the usage of nutritional supplements with carotenoids as a means of managing the condition. Further study on animal models and their neuroprotection mechanisms is nevertheless necessary in light of these findings. (Table 2).
3.1.3. Amyotrophic Lateral Sclerosis
Amyotrophic lateral sclerosis (ALS) is a severe neurodegenerative disease (NDD) characterised by a progressive loss of motor neurons in the ventral horn of the spinal cord, progressive paralysis, and eventually respiratory failure [69, 70]. Depending on inherited genetics, ALS is classified as either regular or family-based. People over the age of 50 to 60 are most commonly affected by sporadic ALS (sALS) [71]. Since the cause of sALS is unknown, it was challenging to identify the genes and causal variables that contribute to the disease. SOD1 mutations, on the other hand, were the main cause of family-based ALS [72]. Enhanced superoxide radical scavenging, adjustments to ATP metabolism, cellular respiration, and post-translational modifications are some of the biological functions of SOD1 [73]. In ALS, the activating superoxide dismutase 1 (SOD1) mutation was linked to the loss of motor neurons, which resulted in neuronal degeneration. Rac1 was immediately activated by SOD1 when it was coupled with endosomes, and NOX was then activated after Rac1 had been activated. Proinflammatory cytokine release was regulated by exosomes (NOX-containing endosomes) via NF-B. NOX played a crucial function in enzymatic regulation, cellular signalling, and antibacterial activity since it was responsible for the formation of free radicals. Rapidly elevating reactive oxygen species (ROS) reduced cellular antioxidant ability and induced DNA damage, apoptosis, and lipid peroxidation. SOD1 mutants, which also cause NOX-driven ROS release, are the cause of motor neuronal death [74]. Misfolded SOD1 proteins were thought to aggregate and cause mitochondrial damage during the aetiology of ALS [75]. Mutant SOD1-related familial ALS disease progression revealed altered signal transduction pathways [76]. (Table 2).
3.2. Cardioprotective
Carotenoids appear to increase the endothelial bioavailability of nitric oxide (NO) by directly removing superoxide anion (O2) from the development of reactive oxygen species (ROS). In light of this, they could be considered a potential oxidant source that regulates endothelial reactions to pro-oxidant or inflammatory stimuli [82]. By oxidising LDL and lowering HDL, certain carotenoids, such as astaxanthin, lutein, and cryptoxanthin, are found to play a greater role in avoiding cardiovascular disease. This can be used to treat cardiac damage [83]. As a function, it can be utilised as a food additive to lessen the heart dysfunction caused by obesity. Additionally, some important studies have been done on humans [84].
3.2.1. Arthrosclerosis
Lycopene, an effective antioxidant, and unsaturated carotenoids can delay the onset of ageing and reduce the risk of cardiovascular disease due to its significant bioactivity [85, 86]. It is a lipophilic molecule that accumulates in the vasculature and human tissues and is transferred into the blood by lipoproteins. It appears to remove ROS and suppress lipid peroxidation and immune system dysfunction [87]. Early carotid atherosclerotic lesions were found to be associated with low plasmatic levels of beta-carotene, lycopene, vitamin A, and E antioxidants [88].
Lutein has been demonstrated to inhibit lipid peroxidation [89] and is well-known for preventing senile cataracts and age-related macular degeneration (AMD) [90, 91]. Oxidative stress is a major risk factor [92]. In actuality, lutein has a potent ability to lessen ROS [93, 94], which prevents the breakdown of the inhibitor kB as well as the activation of the common nuclear transcription factor NF-kB, which is important in many pathogenic reactions (I-kB) [95, 96]. I-kB can translocate into the nucleus when lutein separates it from the NF-kB complex, which reduces the production of signs of inflammation, such as cytokines, chemokines, and iNOS. In addition to lowering levels of monocyte chemotactic protein 1 (MCP-1), TNF-alpha, interleukin 6 (IL-6), prostaglandin 2 (PGE-2), and macrophage inflammatory protein 2 (MIP-2), lutein similarly has a beneficial effect on oxidative stress [97]. (Table 3)
S.No. | Carotenoids | Disease | Neuroprotection Effect | Species | Interference | References |
---|---|---|---|---|---|---|
1 | • Lutein• Lycopene | Parkinson Disease |
![]() ![]() |
Sprague Dawley rat | Reduce brain lesionsRestored the dopamine and acetyline cholinesterase level. | [77, 78] |
2 | • Lutein• Crocetin | Alzheimer Disease |
![]() ![]() |
Wistar rat | Proteolytic dysregulation of Lutein and crocetin. | [79, 80] |
3 | • Lutein | Huntington Disease |
![]() ![]() |
Sprague Dawley rat | Oxidative marker and Neuronal cell death. | [81] |
S.No. | Carotenoids | CVD Effect | Species | Interference | References |
---|---|---|---|---|---|
1 | • Astaxanthin |
![]() ![]() |
Wistar rat | Reduce blood pressure and heart attack risk | [112] |
2 | • β Carotene |
![]() ![]() ![]() |
Sprague Dawley Rat | Reduced LDL level | [113, 114] |
3 | • Lutein• Zeaxanthin | AtherosclerosisHypertension![]() |
Sprague Dawley RatGuinea pig | Protective against AtherosclerosisOxidised glutathioneIntracellular reduced glutathione | [115] |
4 | • Lycopene |
![]() ![]() ![]() |
Sprague-Dawley-Wistar ratRabbit | Beneficial effect of higher serum and tissue levels of lycopene | [116, 117] |
Several preclinical studies have demonstrated that, through triggering the nuclear transcriptional factor Erythroid 2-Associated Protein 2 (Nrf2) and raising phase II biotransformation enzymes, as well as the expression of its antioxidant target genes, astaxanthin also has an indirect antioxidant impact [98-100]. A study with a model of Astaxanthin supplementation significantly reduced the development of myocardial infarction, cardiac dysfunction, and cardiomyocyte death in rats after coronary microembolisation, which was linked to controlling oxidative stress through activating Nrf2/heme oxygenase-1 signalling [101-104].
Recent studies showed that lutein may prevent the progression of atherosclerosis due to its significant antioxidant and anti-inflammatory actions on aortic tissue and the inverse correlation between plasmatic lutein and oxidised LDL [105]. Multiple studies indicate that lutein serum levels, compared to controls, were significantly lower in atherosclerosis and that they were negatively correlated with carotid stiffness [106]. The study showed that increased lutein (such as beta-carotene zeaxanthin) may protect against early atherosclerosis because of the inverse relationship between plasmatic lutein and atherosclerosis [107, 108].
The study by Choi et al. (2011) reported that overweight patients were administered 20 mg of astaxanthin daily for 12 weeks. After the end of the food intervention, they observed a drop in the serum levels of LDL cholesterol and apolipoprotein B. In contrast, there was no difference in the levels of apolipoprotein A1, triglycerides, HDL cholesterol, or total cholesterol. This study supported astaxanthin's claims to have antioxidant effects. It was established that taking that supplement led to lower levels of malondialdehyde and isoprostane and higher levels of superoxide dismutase [109, 110].
The study reported by A D'Odorico et al. 2000 observed to assessed the relationship between plasma levels of carotenoids (α- and β- carotene, lutein, lycopene, zeaxanthin, β-cryptoxanthin), vitamins A and E, and atherosclerosis in the carotid and femoral arteries. This study involved a randomly selected population sample of 392 men and women aged 45–65 years. Carotid and femoral artery atherosclerosis were assessed by high-resolution duplex ultrasound. α- and β- carotene plasma levels were inversely associated with the prevalence of atherosclerosis in the carotid and femoral arteries and with the 5-year incidence of atherosclerotic lesions in the carotid arteries. Atherosclerosis risk gradually decreased with increasing plasma α- and β-carotene concentrations. No associations were found between vitamin A and E plasma levels and atherosclerosis [111].
3.3. Antioxidant Activity
The antioxidant capabilities of carotenoids, which act as primary scavengers of ROS, such as single molecules of oxygen (O2) and peroxyl radicals, are primarily responsible for the positive benefits. Due to the existence of functional groups with increasing polarity and the quantity of conjugated double bonds, carotenes exhibit various antioxidant properties [118].
The study reported by JK Bassett et al. (2016 prospectively examined the relationship between dietary intakes of methionine and B vitamins associated with one-carbon metabolism and the risk of lung cancer. 514 men and women aged 40–69 years, 595 men and 22451 women, for an average of 15 years for lung cancer in current smokers, dietary intake of riboflavin was inversely associated with lung cancer risk. No associations were found for former or never-smokers or dietary intake of any other B vitamins or methionine [119, 120]. The most common carotenoid in the human retina and brain is lutein, a xanthophyll [121]. Age-related macular degeneration can be prevented by taking a supplement containing the vitamins C, E, α-carotene, and zinc, according to the Age-Related Eye Disease Study (AREDS) (AMD). Lutein and zeaxanthin were effective in lowering this risk. (Farouk et al., 2021) [122]. Furthermore, given that age-related macular degeneration is associated with higher levels of oxidative stress and inflammation. Supplementing with lutein may enhance visual function due to its antioxidant properties [123]. Carotenoids have antioxidant properties but can also activate endogenous antioxidant enzymes to prevent DNA damage and shield cells from the oxidative stress brought on by specific stimuli. In rats with norepinephrine-induced heart hypertrophy, superoxide dismutase (SOD) and glutathione peroxidase activities are increased by crocetin, a pharmacologically active metabolite of Crocus sativus L. Another Crocus sativus L. component, crocin, has similarly been demonstrated to boost SOD activity to stop PC-12 cells from dying when they are denied glucose or serum [124, 125]. Recent studies have shown that by triggering the antioxidant network, which includes SOD and catalase, marine carotenoids such as astaxanthin and fucoxanthin also show antioxidant characteristics (Galasso et al., 2018) [126, 127]. In addition to its antioxidant activity, β-cryptoxanthin protects human cells from H2O2-induced damage by stimulating DNA oxidation-related damage repair [128]. Lycopene and beta-carotene also guard against DNA degradation in decreased quantities. However, contrary effects have been observed at higher concentrations in cells that have suffered from oxidative damage. Clinical studies showed a significant reduction in the cardiovascular risk markers of oxidative stress and inflammation, as well as an important improvement in blood.
3.4. Bone Protective
Bone health may benefit from beta-cryptoxanthin, an asymmetric xanthophyll. β-cryptoxanthin may control bone homeostasis, according to rat in vitro experiments and epidemiological data [129].
Through osteoblast stimulation, β -cryptoxanthin stimulates the production of new bone while inhibiting the activity of osteoclasts [130]. Transcriptional regulation may be the mechanism. In β-cryptoxanthin, osteoblasts may alter the expression of bone-forming protein genes and mineralisation [131]. To prevent osteoporosis, β-cryptoxanthin may be crucial for maintaining the balance of bones, especially in postmenopausal women [132].
The study reported by Mackinnon et al. (2016) showed an increase in a clinically relevant bone resorption marker, the crosslinked N-telopeptide of type 1 bone biomarker (NTx), as well as oxidative stress markers in postmenopausal women after a one-month restriction of lycopene in the diet. This also led to a drastic reduction in serum lycopene along with other carotenoids such as α-carotene, β-carotene, lutein, and zeaxanthin [133]. (Table 4).
The study reported by Cristina Russo et al. 2020 observed the effects of lycopene on osteoblast cells, bone mineral density, and bone turnover markers in postmenopausal women. lycopene on the Wnt/β-catenin and ERK 1/2 pathways, RUNX2, alkaline phosphatase, RANKL and COL1A of Saos-2. Lycopene 10µM resulted in higher β-catenin and phERK1/2 protein Vs the vehicle RUNX2 and COL1A mRNA was induced by both 5 and 10 µM doses while RANKL mRNA was reduced. A significant bone density loss was not detected in women taking the tomato sauce while the control group had bone loss. Tomato intake resulted in a greater bone alkaline phosphatase reduction than the control. Lycopene activates the WNT/β-catenin and ERK1/2 pathways, upregulates RUNX2, alkaline phosphatase, COL1A, and downregulates RANKL Saos-2. These processes contributed to preventing bone loss in postmenopausal women [134].
S.No. | Carotenoids | Skin Effect | Species | Inference | References |
---|---|---|---|---|---|
1 | β-carotene |
![]() ![]() ![]() |
SpragueWistar rat |
![]() |
[136, 137] |
2 | Lutein & zeaxanthin |
![]() ![]() |
Wistar Sprague rat |
![]() |
[138, 139] |
3 | Astaxanthin | Reduced alveolar bone![]() ![]() |
SpragueDawley rat | Improved bone mineral density and bone microarchitectureof trabecular bone in the tibia and femur | [140] |
4 | Lycopene | Bone fracture![]() |
Wistar rat | Reduced expression of AGE, RAGE, cathepsin K, and NF-BP65 in the femurs and tibias of obese mice | [141] |
S.No. | Carotenoids | Skin Effect | Species | Inference | References |
---|---|---|---|---|---|
1 | • β-carotene |
![]() ![]() |
WistarSprague rat | PhotoprotectionInpatient | [144-158] |
2 | • Lutein• Zeaxanthin |
![]() ![]() |
WistarSprague rat | Protect against UVR-induced skin damage | [12, 146] |
3 | • Astaxanthin |
![]() |
Sprague rat | UVA-induced MMP-1 and skin fibroblast elastase | [147] |
4 | • Lycopene |
![]() |
Sprague rat | Protect acute & potentially of photodamage | [148] |
The study reported by Z-Q.Zhang et al. 2016 observed a relationship between serum carotenoids and bone mineral density (BMD) in the Chinese population. They found that women with higher serum β-cryptoxanthin, lycopene, or α-carotene exhibited higher BMD at various bone sites. They measured 1898 women and 933 men aged 59.6 years who completed serum β-cryptoxanthin, zeaxanthin, lutein, lycopene, and α-carotene concentration analyses and BMD assessments. Women in the top (vs. bottom) quartiles of serum β-cryptoxanthin, lycopene, or α-carotene exhibited 1.8–2.3, 1.5–2.0, or 1.3–2.7% higher BMD at the bone sites with significant results, respectively. For men, the corresponding values were 2.6–4.0% for α-carotene at the whole body and hip regions. These results suggest that serum carotenoids have a favorable association with bone health [135].
3.5. Skin Protective
A healthy diet contains many carotenoids, which build up in the skin and effectively shield it against sunburn, aging, and damage caused by UV rays. Carotenoids have a great capacity to scavenge reactive oxygen species, such as singlet oxygen molecules or peroxide radicals, due to their distinctive structure with ten or more conjugated double-bonds (Table 5) [88, 142].
The study reported by M. Rizwan et al. 2016, observed that tomato paste – rich in lycopene, a powerful antioxidant can protect human skin against UVR‐induced effects partially mediated by oxidative stress. 20 healthy women (median age 33 years, range 21–47; phototype I/II) ingested 55 g tomato paste (16 mg lycopene) in olive oil, or olive oil alone, daily for 12 weeks. Pre and post-supplementation, UVR erythemal sensitivity was assessed SD erythemal D30 was significantly higher following tomato paste vs. control, while the MED was not significantly different between groups of tomato paste. Pre-supplementation, UVR induced an increase in MMP 1 Tomato paste containing lycopene providing protection against acute and potentially longer-term aspects of photodamage [143].
3.6. Weight Management
The carotenoid metabolites can control transcription through various nuclear hormone receptor superfamily members, such as retinoic acid receptors. Additionally, carotenoids and their byproducts may control pathways like NF-b or Nrf-2. Last but not least, the antioxidant capacity of carotenoids may also lessen the overall oxidative burden or prevent its accumulation, positively impacting obesity and weight management [149].
In the study Phichittra Od Ek of β-carotene reported in rats, papaya was observed to lower the expression of carotenoid, inflammatory, and oxidative stress markers. 28 male Sprague Dawley rats were given an HF diet for 12 weeks to make them obese. (Table 6). According to this study's findings, papaya may be able to reduce the risk of obesity brought on by adiposity [150].
S.No. | Carotenoids | Weight Effect | Species | Inference | Reference |
---|---|---|---|---|---|
1 | • β-carotene |
![]() ![]() |
SpragueWistar rat | Increased the MRNA astrogen's mRNA level is influenced by the insulin-like growth factor-1 (Igf-1) concentration. | [152] |
2 | • Astaxanthin |
![]() |
WistarSprague Dawley rat |
![]() |
[153] |
3 | • Lutein & zeaxanthin |
![]() ![]() |
SpragueWistar rat | Inducing AMPK activation, inhibiting lipogenesis and lowering the size and weight of adipocytes and their intracellular lipid content | [154] |
4 | • Lycopene |
![]() ![]() ![]() |
SpragueWistar rat | Reduced the Oxidative stress in Lycopene | [155] |
The study reported by JA Canas et al. (2017) compared the effects of mixed-carotenoid supplementation (MCS) versus placebo on adipokines and the accrual of abdominal adiposity weight in children with obesity. Twenty (6 male and 14 female) children with simple obesity [body mass index (BMI) > 90%], a mean age of 10.5 ± 0.4 years. β-carotene showed an inverse correlation with BMI z-score, waist-to-height ratio, visceral adipose tissue, and subcutaneous adipose tissue (SAT) at baseline. MCS increased β-carotene, total adiponectin, and high-molecular-weight adiponectin compared with placebo. The decrease in BMI z-score, waist-to-height ratio, and SAT and the concomitant increase in the concentration of β-carotene and high-molecular-weight adiponectin by MCS suggest the beneficial role of MCS in children with obesity [151].
3.7. Immune Protective
In immune protection in humans with cardiovascular disease, β-carotene and other carotenoids have an antagonistic relationship with inflammatory markers and immunological activation. In contrast to healthy controls, CAD patients had increased immunological activity (soluble interleukin 2 receptor, CRP, CD4+/CD25+ cells), whereas their plasma levels of β-carotene and lycopene were significantly lower [156].
A clinically significant decrease in morbidity and mortality in children is correlated with increased vitamin A intake and better vitamin A [157].
3.7.1. Retinoic Acid
Cytochrome P450 26 metabolises retinoic acid (RA) (CYP26) [158]. After intake, retinol can be converted to retinaldehyde (retinal) by widely distributed alcohol dehydrogenases (ADH), which is subsequently converted into RA by retinaldehyde/aldehyde dehydrogenases (ALDH) in the liver. Additionally, the gut-associated lymphoid tissue expresses (GALT) [159, 160]. Non-provitamin A carotenoids are active in immunological regulation, although RA is the main active metabolite impacting the immune system [161].
Preclinical studies reported by Kiko Tet. et al. 2012 suggest that Retinoic acid (RA) controls the expression of molecules that are gut-homing by dendritic cell (DC) precursors in the bone marrow. Through the induction of regulatory T cells (Treg), these cells move in the gut and promote oral tolerance. The chemokine receptor CCR7+ DC that RA also generates moves to the mesentery lymph nodes (MLN) and causes gut homing in T cells. Numerous local signals control how much RA the DC produces. ALDH expression in DC is induced by microbial signals, including butyrate generated by commensal bacteria and Toll-like receptor (TLR) 2 and TLR5 signals. In addition to IL-4 from Th2 and ILC2 (innate lymphoid cells), TGF- (transforming growth factor beta) may also promote the (Aldehyde dehydrogenase appearance of ALDH [162]. (Table 7)
S.No. | Carotenoids | Skin Effect | Species | Inference | Reference |
---|---|---|---|---|---|
1 | • β-carotene |
![]() |
Wistar rat | Inhibit tumor Growth raises blood levels of NK, IL-2, TNF-, WBC, TP, and ALBA/G. | [164] |
3 | • Lutein & zeaxanthin |
![]() |
WistarSprague rat | Ameliorated the oxidative damage by reducing MDA![]() |
[165] |
4 | • Lycopene |
![]() |
Wistar rat | Lipid peroxidation and DNA damage | [166] |
S.No. | - | Carotenoids | Cancer Effect | Species | Inference | References |
---|---|---|---|---|---|---|
1 | Prostate cancer | • β-carotene• Lycopene |
![]() ![]() |
WistarSprague rat | Reduced Prostate cancer risk.Slight induction of a few genes affecting proliferation and apoptosis. | [177] |
2 | Breast Cancer | • Beta carotene |
![]() |
SpragueWistar rat |
![]() ![]() |
[178] |
3 | Lung Cancer | • Beta carotene |
![]() |
SpragueWistar rat | Higher risk of developing lung cancer. | [179] |
4 | Colorectal cancer | • Lycopene |
![]() |
SpragueWistar rat | Proliferation in H-29 colon cancer cell. | [180] |
5 | Pancreatic cancer | • Lycopene• Beta carotene• Beta cryptoxanthin |
![]() |
SpragueWistar rat | Reduced pancreatic cancer. | [181] |
6 | Gastric cancer | • Beta carotene• Lycopene |
![]() ![]() |
SpragueWistar rat | Reduction of gastric cancer growth. | [182] |
Some preclinical studies on carotenoids suggest that they may impact humoral immunity. IgA concentrations and the quantity of ASC in the jejunum were elevated in mice after receiving 50 mg/kg of carotene for 21 days [163]. Additionally, in rats, β-cryptoxanthin (5–10 mg/kg, 14 and 21 d), IL-4, elevated blood CD4, and humoral immunity (IgG, IgM, and IgA) [161]
3.8. Cancer Protective
Several studies show the efficacy of carotenoids in preventing cancer. The majority of the time, cell cycle arrest caused by carotenoids is linked to reduced expression of cyclin D1, CDK4, CDK6, and cyclin D1. As a result, it also increases GADD45, which prevents cells from entering the S phase [167]. However, substances like crocetin and crocin isolated from saffron demonstrated anti-metastasis qualities such as anti-invasiveness, anti-migration, and anti-non-adhesive effects when combined with the 4T1 cell line in breast cancer [168]. Lycopene and β-cryptoxanthin are two carotenoids that have been identified to block the NF-B signalling pathway, which is useful in treating lung and prostate cancer. Evidence shows -carotene has anti-angiogenic properties [169-171]. (Table 8) Higher serum levels of α-carotene, cryptoxanthin, and lycopene, in particular, have been linked to a decreased risk of lung cancer in humans [172, 173]. Additionally, carotenoids 1) serve as antioxidants, 2) are retinoic acid precursors (RA) [174, 175], 3) promote gap junction communication,4) boost immunity, restrict cell proliferation, 6) promote cell apoptosis, 7) upregulate insulin-like growth factor-binding protein (IGFBP) to reduce insulin-like growth factor 1 (IGF-1)-stimulated cell proliferation, and 8) upregulate carcinogen-metabolising enzymes [176].
CONCLUSION
Carotenoids are naturally occurring, fat-soluble, highly unsaturated yellow, red, and orange pigments found in plants, fungi, bacteria, and algae. The amount of carotenoids present is typically correlated with the intensity of colour. Vegetables and fruits naturally contain large amounts of carotenoids. Due to their chemical constitution and interactions with cellular membranes, carotenoids have biological features that make them antioxidants. Lycopene, astaxanthin, fucoxanthin, and lutein, in particular, are effective FRs, quenchers of ROS and NOS; therefore, their antioxidant and anti-inflammatory action may assist in reducing cardiovascular risk factors such as inflammatory indicators, hyperlipidemia, insulin resistance, and obesity. Data shows they improve cardiovascular health, cognitive function, and eye health. They may also lower the risk of several cancers. This is particularly problematic for lutein since its health benefits include slowing the course of age-related macular degeneration. Carotenoids are naturally occurring lipophilic secondary metabolites with significant anti-NDD therapeutic potential. The several natures of carotenoids and their potential as therapeutic agents in reducing or preventing the mechanisms and studies of neurodegeneration in different diseases like Alzheimer's, Parkinson's, stroke, etc. The outcomes of human studies showed decreased levels of carotenoids in the plasma of neurodegenerative patients. At this point, human studies have primarily focused on a statistical analysis of connections between consumption and blood carotenoid levels on the one hand and lowered risk of disease progression on the other. However, studies on human subjects showed the beneficial impact of carotenoids against NDD, consistent with the findings from cell culture and animal studies.
LIST OF ABBREVIATIONS
NDDS | = Neurodegenerative disease |
ASTA | = Astaxanthin |
IPP | = Isopentenyl diphosphates |
DMAPP | = Dimethylallyl diphosphate |
PSY | = Phytoene synthase |
PDS | = Phytoene desaturase |
CRTISO | = Carotene isomerase |
LCY | = Lycopene cyclase |
LCYB | = Lycopene cyclase β- ionone |
LCYB | = Lycopene cyclase ε- ionone |
CVD | = Cardiovascular Disease |
PD | = Parkinson Disease |
SNPc | = Substantia nigra pars compacta |
ROS | = Reactive oxide species |
PINK1- PTEN | = induced putative kinase 1 |
GSH | = Glutathione |
AD | = Alzheimer's disease |
NFT | = Neurofibrillary tangle |
SOD1 | = Superoxidase dismutase 1 |
NOX | = Nitric oxide |
LDL | = Low-density level |
HDL | = High-density level |
AMD | = Age-related muscular degeneration |
MIP2 | = Microphage inflammatory protein |
NrF2 | = Nuclear transcriptional factor Erythroid 2-Associated Protein 2 |
AREDS | = Age-Related Eye Disease Study |
ADH | = Alcohol dehydrogenase |
ALDH | = Aldehyde dehydrogenase |
GADH | = Gut-associated lymphoid tissue |
RA | = Retinoic acid |
MLN | = Mesentery lymph nodes |
TLR | = Toll-like receptors |
IL-2 | = Interleukin-2 |
TNF | = Tumor necrosis factor |
MDA | = Malondialdehyde |
HFD | = High-fat diet |
CONSENT FOR PUBLICATION
Not applicable.
AVAILABILITY OF DATA AND MATERIALS
The authors confirm that the data supporting the findings of this study are available within the article.
FUNDING
None.
CONFLICT OF INTEREST
The authors declare no conflict of interest, financial or otherwise.
ACKNOWLEDGEMENTS
I would like to express my special thanks of gratitude to my Guide “Dr. Pranay Wal,” and all faculty members and PSIT-Pranveer sigh Institute (Pharmacy) for their able guidance and support in completing my review article.