All published articles of this journal are available on ScienceDirect.
Effects of Caraway and (S)-(+)-carvone Extract on the Expression of Genes Coding Key Glycolytic Enzymes in Streptozotocin-induced Diabetic Rats
Abstract
Background:
Diabetes mellitus is a metabolic disorder causing dysfunctional regulation of carbohydrate metabolism and contributing to multiple serious health challenges worldwide.
Aims:
This research explores the effect of caraway hydroalcoholic extract and (S)-(+)-carvone on the transcription of genes coding for key glycolytic enzymes in the liver of the diabetic rat treated with streptozotocin (STZ).
Methods:
In this experimental study, diabetes was established in four groups of rats by injecting 45 mg/kg of STZ intraperitoneally. Further normal rats that were not injected formed the control group. Over four weeks, the effects of caraway hydroalcoholic extract (150 and 250 mg/kg) and carvone (100 mg/kg) were evaluated using PCR and histopathological evaluation, specifically in the form of effects on the transcription process of genes coding key glycolytic enzymes in the liver of the diabetic rats.
Results:
Over the four-week assessment period, rats’ food intake, and therefore blood glucose levels, were decreased through the daily oral administration of carvone and caraway extract (150 mg/kg) when compared to those administered with further STZ. The expression of key glycolytic enzymes, including glucokinase, pyruvate kinase, and phosphofructokinase 1 in the liver of diabetic rats was restored to near-normal levels by carvone and caraway extract, especially at the 150 mg/kg dose. Histopathological evaluation of diabetic rat liver demonstrated that administration of caraway extract and carvone decreased the STZ-induced damage to liver tissue.
Conclusion:
150 mg/kg of caraway extract strongly regulates glycolysis by regulating the gene expression of key glycolytic enzymes in diabetic rats.
1. INTRODUCTION
Diabetes is a metabolic disease characterized by an insulin deficiency and/or insulin insensitivity, leading to the cell’s inability to utilize carbohydrates as energy, consequently increasing blood sugar levels [1]. Failure to maintain glycemic regulation and histopathological changes occur in a range of tissues such as the pancreas, liver, and kidney, contributing to multiple micro and macrovascular complications such as cardiovascular disease, renal disease, neuropathies, and retinopathies, as well as many others. As such, treatment focused on improving glucose metabolism is needed to improve diabetes management and as such, to reduce hyperglycemia and delay the development and progress of diabetic complications [2, 3].
The principal hormone of glucose homeostasis is insulin. This hormone binds to insulin receptors throughout the body, triggering signaling, which regulates glycogen synthesis and the control of glucose metabolism. The metabolism of glucose through the involvement of insulin can increase the abundance of glucokinase (GK), pyruvate kinase (PK), and phosphofructokinase 1 (PFK-1) gene expression, which in turn enhances glucose utilization in the hepatocytes to create and maintain homeostasis [4, 5].
The main type of treatment for diabetes and controlling the associated hyperglycemia is in the form of insulin which primarily focuses on lowering and maintaining blood glucose levels. As a better understanding of the pathogenesis and complexity in treating the disease arises, so too must the need for the development of more effective and safer drugs to treat the disease. Recently, there has been widespread traditional use in several cultures of decoctions prepared from medicinal plants in the treatment of diabetes. There are several possible mechanisms by which medicinal plants induce hypoglycemia. These included enhancing regeneration or revitalization of damaged pancreatic beta cells, and protecting against further damage, enhancing insulin synthesis and secretion from the beta-cells, decreasing glucose absorption from the gastro-intestinal system, increasing insulin sensitivity of the tissues, possessing insulin mimicking effects, and changing the activity of some enzymes involved in glucose metabolism. In recent years, there has been renewed interest in plant medicine for the treatment of different diseases as herbal drugs are generally out of toxic effect reported from research work conducted on experimental model animals [6-10].
The use of the natural herb caraway (Carum carvi L.) in diabetes therapy is thought to contribute to improved therapeutic efficacy, and as such, efforts are being made to discover the bioactive compounds responsible for this effect. The known main constituents of caraway have been demonstrated to be carvone (40–60%), limonene, carveol, dihydrocarveol, thymol, in addition to glucosides and flavonoids. The caraway extract seeds had an increasing effect on basal plasma insulin concentrations in diabetic rats. It appears that the seed extracts of these plants exert a hypoglycemic effect dependent on insulin secretion. Recent-onset insulinopenia in STZ-diabetic rats was associated with glucose overproduction in the basal (hyperglycemic) state. The hypoglycemic activity of this plant may therefore be due to inhibition of hepatic glucose production and/or stimulation of glucose utilization by peripheral tissues, especially muscle and adipose tissue. The plant extracts could also act as inhibitors of tubular renal glucose reabsorption [11-13].
This study aims to evaluate the effects of (S)-(+)-carvone on the transcript abundance of genes coding crucial glycolytic enzymes in rats with diabetes induced by streptozotocin (STZ), in relation to their blood glucose, body weight, and food intake. These observations aim to assess whether caraway hydroalcoholic extract and (S)-(+)-carvone provide an antihyperglycemic advantage by regulating the transcript level of genes coding for GK, PK, and PFK-1 enzymes, all of which are important in glucose homeostasis. We aim to improve the understanding of the mode of action of (S)-(+)-carvone and identify new targets for developing novel therapeutic agents.
2. MATERIALS AND METHODS
2.1. Plant Hydroalcoholic Extract and Chemicals
A 300-gram sample of caraway seed was air-dried, powdered, and soaked in ethanol: water (80:20) solution for 60 minutes. The hydroalcoholic extract was obtained by percolating for three days as described by Mardani et al. [14]. The extract was shaken, filtered, and evaporated under reduced pressure in a rotary evaporator until a concentrated extract was obtained. Finally, this product was freeze-dried to prepare a hydroalcoholic extract. The extraction of (S)-(+)-carvone from caraway seeds was carried out using supercritical fluid extraction as described by Raal et al. [15]. Streptozotocin was obtained from Sigma-Aldrich and stored at 4°C in the dark [16]. All other chemicals were purchased from commercial suppliers.
2.2. Rats’ Preparation
Male Wistar rats (250–160 grams) were obtained from the Rafsanjan University of Medical Sciences, Rafsanjan, Iran. The rats inhabited an air-conditioned animal house at light 300 lx, 55% relative humidity, and 25±1°C, with free access to water and food with a 12 hour light/dark cycle. The rats were monitored carefully and operational procedures were conducted in line with the guidance of the Institutional Animal Ethical Committee (IAEC).
2.3. Induction of Diabetes
The rats were fasted for 16 hours, and then diabetes was induced by an intraperitoneal injection of 45 mg/kg of STZ dissolved in 0.1 M buffer citrate. STZ-injected rats were administered a 5% glucose solution overnight to avoid STZ-associated hypoglycemia. Glucose levels were measured in each rat by taking a blood sample from the cut tip of the rat’s tail and using an Accu-Chek test strip on the third day after injection [17]. Blood glucose levels over 200 mg/dl were considered indicative of diabetes and these rats were selected for the study.
2.4. Experimental Design
Group I rats (n=8) comprised the control condition receiving only water. To form the study groups, 32 diabetic rats were categorized randomly into four further groups, each comprising eight rats. Group II rats were administered further STZ (45 mg/kg) dissolved intragastrically in 1ml corn oil; Group IΙI rats received caraway hydroalcoholic extract (150 mg/kg); Group IV rats received caraway hydroalcoholic extract (250 mg/kg); and Group V rats were given (S)-(+)-carvone (100 mg/kg). Hydroalcoholic extracts of caraway and (S)-(+)-carvone were dissolved in 1ml corn oil, which was administered orally each day for one month, during which blood glucose levels, body weight, and food intake were measured once a week as described by Muruganathan and Srinivasan [17]. Following this, the rats were fasted overnight and terminated in the morning.
2.5. Histopathological Evaluation of the Liver
Liver tissue was fixed in 10% formalin for 48 hours and subjected to various water-ethyl alcohol solutions, rinsed in xylene, and exposed to paraffin. The liver sample (5 mm thick) was prepared and then staining was performed using haematoxylin and eosin dye [18]. Prepared slides were finally used for microscopic observation using xylene medium.
2.6. Gene Expression Evaluation
The RNeasy® Lipid Tissue Mini Kit (Qiagen, Germany) and SuperScript® IV First-Strand Synthesis Kit (Thermo Fisher Scientific, United States) were used to isolate the total RNA from rat liver samples and synthesize the complementary DNA, respectively. Real-time quantitative polymerase chain reaction (PCR) sampling of GK, PK, and PFK-1 genes was conducted using an SYBR Green detector with the BioRad CFX96 system (Bio-Rad Laboratories, United States) (Table 1). Data were analyzed using the ΔΔCT procedure and β-actin was used as the reference gene.
2.7. Statistical Analysis
Continuous variables were expressed as mean±SD and categorical variables as percentages. Analysis of variance (ANOVA) and Duncan’s Multiple Range Test were used to compare data between different rat groups. Statistical analyses were performed using SPSS (version 16.0, SPSS Inc., Chicago, IL, USA). Significance was considered at a threshold of p<0.05.
2.8. Ethical Considerations
The study protocol and procedures were approved by the Laboratory Animal Research Ethics Committee of RUMS (No. IR.RUMS.REC.1398.018) and conducted following the Animal Research: Reporting of in vivo Experiments (ARRIVE) guidelines and the Institutional Animal Ethical Committee (IAEC) guidelines for the care and use of laboratory rats.
3. RESULTS
3.1. Effect of Caraway Extract and (S)-(+)-carvone on Body Weight and Food Intake
Changes in body weight and food intake in the control and treatment groups are presented in (Figs. 1 and 2). Rats treated with further STZ (Group II) exhibited a decrease in body weight despite increased food intake when compared to those of the control group (Group I). Rats administered with either (S)-(+)-carvone or caraway extract (Groups III-V), especially in the dose of 150 mg/kg (Group III), exhibited decreased food intake.
Gene | Primer | Primer sequences 5′ to 3′ |
Glucokinase | Forward | 5'-TGGTGCTTTTGAGACCCGTT-3' |
Reverse | 5'-GAAGCCCCAGAGTGCTTAGG-3' | |
Pyruvate kinase | Forward | 5'-AGAAACAGCCAAAGGGGACT-3' |
Reverse | 5' -CATTCATGGCAAAGTTCACC-3' | |
Phosphofructokinase 1 | Forward | 5'-AGACCTGAAAGCCAACGTGGAG-3' |
Reverse | 5'-GGCCCAGAACGTTGGTCCTA-3' | |
β-actin | Forward | 5'-GTCCACCCGCGAGTACAAC-3' |
Reverse | 5'-GACGACGAGCGCAGCGATA-3' |
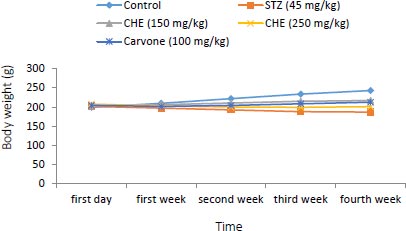
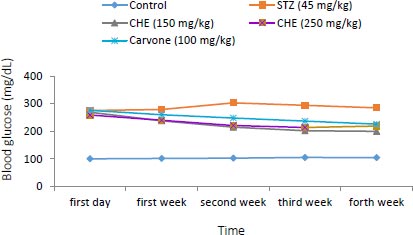
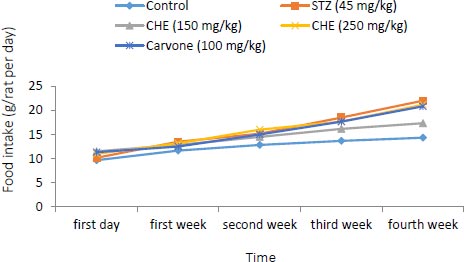
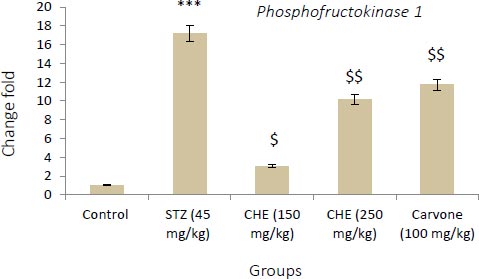
Values shown are the mean ± SD over 5 rats in each group. Key: *** = P<0.001 when compared to the control group. $ = P<0.001 and $$ = P<0.01 when compared to the STZ group.
3.2. Effect of Caraway Extract and (S)-(+)-carvone on Blood Glucose
As shown in Fig. (3), the blood glucose level was raised in diabetic rats administered with STZ compared to those in the control group. Rats administered with (S)-(+)-carvone and caraway extract exhibited decreased levels of hyperglycemia, especially those treated with the 150 mg/kg regime (Group III).
3.3. Effect of Caraway Extract and (S)-(+)-carvone on the Transcript Level of Genes Coding Key Glycolytic Enzymes
Figs. (4-6) display changes in the transcript abundance of genes coding key glycolytic enzymes in the hepatocytes of rats. The expression of GK, PK, and PFK-1 genes increased significantly in diabetic rats treated with STZ when compared to those in the control group. Rats treated with (S)-(+)-carvone and caraway extract exhibited nearer-normal expression of these genes.
3.4. Effect of Caraway Extract and (S)-(+)-carvone on Liver Histology
Histopathological observations showed normal liver sections in the non-diabetic control group (Group I) rats (Fig. 7). STZ-treated rats displayed changes in hepatic parenchyma by way of dilatation in the portal and central veins. Caraway extract and (S)-(+)-carvone significantly improved these degenerative changes in the rats.
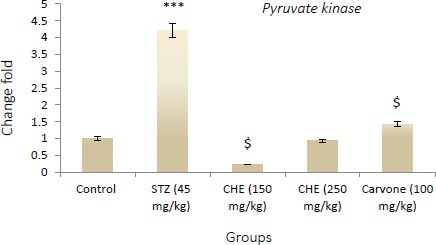
Values shown are the mean ± SD over 5 rats in each group. Key: *** = P<0.001 when compared to the control group. $ = P<0.001 and $$ = P<0.01 when compared to the STZ group.
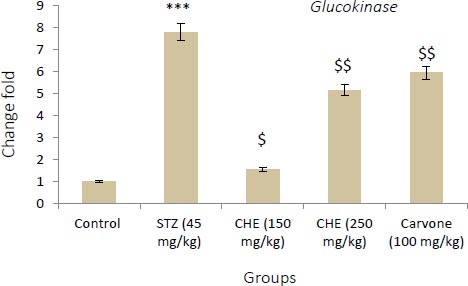
Values shown are the mean ± SD over 5 rats in each group. Key: *** = P<0.001 when compared to the control group. $ = P<0.001 and $$ = P<0.01 when compared to the STZ group.
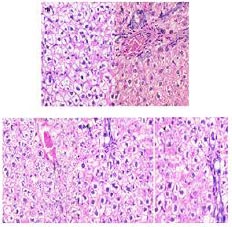
4. DISCUSSION
Streptozotocin-derived hyperglycemia in rats has been suggested as a suitable model for the primary screening of bioactive compounds against diabetic complications [19]. Insulin is the hormone that acts as a primary modulator for glucose homeostasis in blood by promoting the uptake of glucose by cells, including hepatocytes [20, 21]. Normalizing hyperglycaemia is the primary desired outcome for the management of diabetes mellitus, aiming to limit the chronic exposure of tissue to the hyperosmolar effects of high glucose levels, which can result in long-term micro and macrovascular complications [22]. Consistent with this, the present study shows an incremental increase in blood glucose levels in streptozotocin-treated rats. With respect to demonstrating the potential for these preparations to be used in the treatment of diabetes mellitus, this study has also shown that these increased blood glucose levels were restored by the administration of caraway hydroalcoholic extract and [S)-(+)-carvone to levels similar to those seen in the control rats.
Findings of previous studies have suggested that carvone possesses anti-hyperglycemic properties that are induced by improving insulin secretion in streptozotocin-treated rats [23]. In a study, the hypoglycemic effect of caraway ethanolic extract was investigated in normal and streptozotocin-induced diabetic rats. The results showed that the caraway ethanolic extract seeds at doses 0.2, 0.4 and 0.6 g/kg body weight significantly decreased serum glucose in diabetic rats in 3 and 5 h, but not in healthy rats [24]. In another study, single dose or 14 days of oral administration of the aqueous extracts (20 mg/kg) produced a significant decrease in blood glucose levels in STZ diabetic rats (P<0.001); the blood glucose levels were nearly normalized 2 weeks after daily repeated oral administration of aqueous extracts (20 mg/kg) (P<0.001). No highly significant changes on blood glucose levels were noticed in normal rats after both acute and chronic treatments with extract [25]. The hypoglycaemic activity of caraway may therefore be due to the inhibition of hepatic glucose production and/or stimulation of glucose utilisation by peripheral tissues, especially muscle and adipose tissue. The caraway extracts could also act as inhibitors of tubular renal glucose reabsorption [11-13].
In the present study, diabetic rats exhibited increased food intake in comparison to control rats, which may be due to the inaccessibility of carbohydrates needed to produce energy within cells, due to the metabolic dysregulation of insulin [26]. The treatment of these diabetic rats with caraway hydroalcoholic extract or (S)-(+)-carvone was associated with decreased weight gain when compared to the STZ rats, and, therefore may indicate the potential for the use of these extracts in future diabetes management options.
The genes coding for key glycolytic enzymes, such as GK, PK, and PFK-1 genes [27], were significantly increased in the hepatocytes of diabetic rats in the present study. The enzyme glucokinase (GK) is responsible for modulating the level of blood glucose and sustaining metabolic processes [28]. The crucial function of this enzyme in modulating glucose has made it a promising target for the treatment of diabetes mellitus [29]. Pyruvate kinase (PK) catalyzes the last stage of the glycolysis process, which generates pyruvate and a second ATP molecule [30, 31]. Phosphofructokinase-1 (PFK-1) catalyzes the conversion of ATP and fructose 6-phosphate to ADP and fructose 1,6-bisphosphate [32, 33]. In the present study, analysis of the expression of these three key genes revealed a significant decrease in the transcription level of GK, PK, and PFK-1 genes in the treatment group. Therefore, in diabetic rats with altered blood glucose levels, treatment with caraway hydroalcoholic extract and (S)-(+)-carvone may result in a shift in the transcription abundance of genes coding for enzymes involved in glycolysis, such as GK, PK and PFK-1, resulting in enzyme levels closer to those found in non-diabetic rats.
The novelty of this research is that, for the first time, we have demonstrated that (S)-(+)-carvone and caraway hydroalcoholic extract (especially 150 mg/kg), normalized the expression of genes coding key enzymes for glycolysis in streptozotocin-induced diabetic rats. As the amelioration of hyperglycemia and the expression of GK, PK, and PFK-1 genes are considered to be signs of the successful treatment of diabetes [34-36], this research provides a promising basis for further investigation, namely the use of (S)-(+)-carvone to develop novel anti-hyperglycemic medications.
There are few reports describing the biological activity of carvone [11-13]. However, this research reinforces the understanding of the anti-hyperglycemic properties of this bioactive ingredient, providing evidence for its use in future research aiming to minimize cellular damage caused by hyperglycemia in diabetes.
CONCLUSION
This work demonstrates the anti-hyperglycemic properties of (S)-(+)-carvone and caraway extract in streptozotocin-induced diabetic rats. It is proposed that this effect is achieved through a shift in the transcription abundance of GK, PK, and PFK-1 genes. These findings suggest that 150 mg/kg of caraway extract can be used as a protective agent against hyperglycemia in streptozotocin-induced metabolic disorders, which is promising for future research into therapies for diabetes mellitus.
LIST OF ABBREVIATIONS
STZ = | Streptozotocin |
GK = | Glucokinase |
PK = | Pyruvate kinase |
PFK-1 = | Phosphofructokinase 1 |
IAEC = | Institutional Animal Ethical Committee. |
AUTHORS’ CONTRIBUTIONS
MH, MMah were responsible for the study concept and design. RZ, MNK, MH, RH, MMah led data collection. RZ, RH, MMah were responsible for the analysis and interpretation of data. MNK wrote the first draft. MH, SZ, RH, and MMah contributed to the writing of the second and third draft. MNK, MH, RSD, AW, RH, and MMah provided comments on initial drafts and coordinated the final draft. AW edited drafts of the manuscript. All authors read and approved the final manuscript. All authors take responsibility for the integrity of the data and the accuracy of the data analysis.
ETHICS APPROVAL AND CONSENT TO PARTICIPATE
The animal study protocol and procedures were accepted by the Laboratory Animal Research Ethics Committee of RUMS (No. IR.RUMS.REC.1398.018).
HUMAN AND ANIMAL RIGHTS
No humans were used that are the basis of this study. All the animal experiments were conducted as per the Institutional Animal Ethical Committee (IAEC) guidelines for the care and use of laboratory rats and arrive guid lines.
AVAILABILITY OF DATA AND MATERIALS
The data used in this study are available from the corresponding author on request.
FUNDING
This project was supported by grant number 9/991 from the Rafsanjan University of Medical Sciences.
ACKNOWLEDGEMENTS
Authors take this chance to thank the Molecular Medicine Research Center, Rafsanjan University of Medical Sciences, for its warm cooperation.